Back to Journals » Journal of Multidisciplinary Healthcare » Volume 17
Research Progress on the Correlation Between Hypertension and Gut Microbiota
Authors Cui X , Zhang T, Xie T, Guo FX, Zhang YY, Deng YJ, Wang Q, Guo YX, Dong MH , Luo XT
Received 12 February 2024
Accepted for publication 23 April 2024
Published 16 May 2024 Volume 2024:17 Pages 2371—2387
DOI https://doi.org/10.2147/JMDH.S463880
Checked for plagiarism Yes
Review by Single anonymous peer review
Peer reviewer comments 2
Editor who approved publication: Dr Pavani Rangachari
Xiaomei Cui,1,2 Ting Zhang,2 Tao Xie,2 Fang-xi Guo,2 Yu-ying Zhang,2 Yuan-jia Deng,2 Qi Wang,1,2 Yi-xing Guo,1,2 Ming-hua Dong,1,2 Xiao-ting Luo1,3
1Key Laboratory of Cardio Cerebrovascular Diseases, Ministry of Education, Gannan Medical University, Ganzhou, People’s Republic of China; 2School of Public Health and Health Management, Gannan Medical University, Ganzhou, People’s Republic of China; 3School of General Medicine, Gannan Medical University, Ganzhou, People’s Republic of China
Correspondence: Xiao-ting Luo, Golden Campus of Gannan Medical University, Zhanggong District, Ganzhou City, Jiangxi Province, People’s Republic of China, Tel +86 13677975578, Email [email protected]
Abstract: Among cardiovascular diseases, hypertension is the most important risk factor for morbidity and mortality worldwide, and its pathogenesis is complex, involving genetic, dietary and environmental factors. The characteristics of the gut microbiota can vary in response to increased blood pressure (BP) and influence the development and progression of hypertension. This paper describes five aspects of the relationship between hypertension and the gut microbiota, namely, the different types of gut microbiota, metabolites of the gut microbiota, sympathetic activation, gut–brain interactions, the effects of exercise and dietary patterns and the treatment of the gut microbiota through probiotics, faecal microbiota transplantation (FMT) and herbal remedies, providing new clues for the future prevention of hypertension. Diet, exercise and traditional Chinese medicine may contribute to long-term improvements in hypertension, although the effects of probiotics and FMT still need to be validated in large populations.
Keywords: blood pressure, metabolites, gut-brain, treatment
Graphical Abstract:
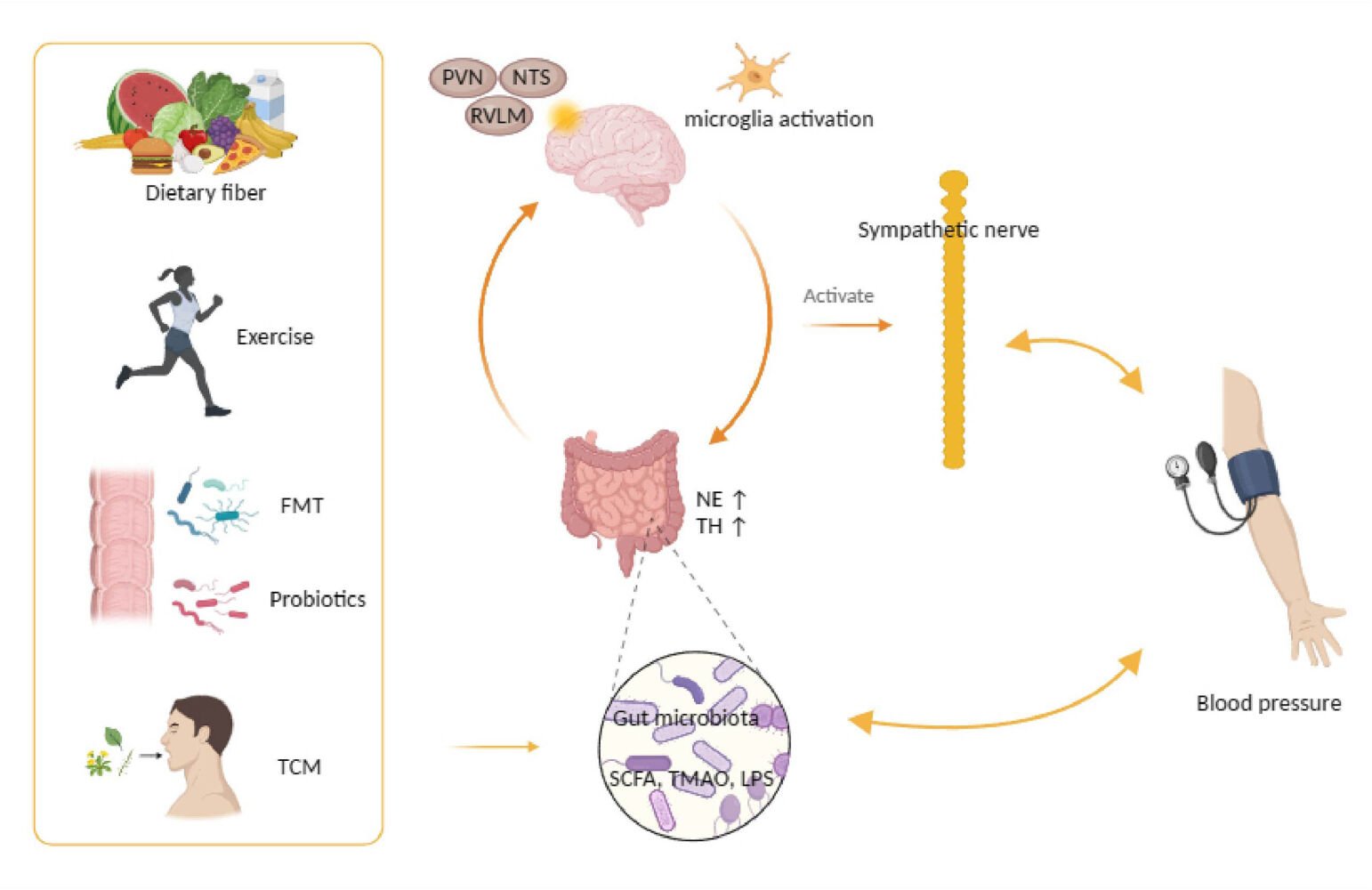
Introduction
Cardiovascular disease (CVD) has been a major threat to global health during the last 30 years. Hypertension is the primary risk factor for CVD and has become a global public health crisis.1,2 High SBP (systolic blood pressure) was the top Level 2 risk factor for attributable fatalities in 2019, accounting for 10.8 million (95% uncertainty interval [UI] 9.51–12.1) deaths (19.2% [16.9–21.3] of all deaths in 2019).3 High BP is caused by a complex combination of genetic, nutritional, and environmental variables, although the exact interactions among these factors is yet to be elucidated. To date, the precise reason for the rising incidence rate has not been elucidated. One study demonstrated that only a small portion of hypertension was caused by heredity and a much greater proportion of cases is caused by complex environmental factors.4
Recently, there has been significant interest in the gut microbiota’s potential to affect host health.5–7 There are trillions of microbial cells in the human intestine, predominantly bacteria and viruses. These cells are collectively commonly referred to as gut microbiota and are an important part of our ecosystem.5 The gut is an important site for the absorption and digestion of nutrients and the production of metabolites. Therefore, the daily diet and the nutritional status of the body have a significant impact on the formation of the gut microbiota.8 The gut microbiota is linked to a variety of disorders, including hypertension, atherosclerosis, and type 2 diabetes mellitus (T2DM).9–11 This paper comprehensively examines the complex relationship between the gut microbiota and hypertension, providing a diverse perspective on the treatment of hypertension by analysing the effects of various types of gut microbiota, gut microbiota metabolites, sympathetic activation and gut-brain interactions, and exercise and dietary patterns, as well as the positive effects of probiotics, FMT, and traditional Chinese medicine (TCM) on BP. The aim of this paper is to offer a comprehensive and systematic review of the latest scientific developments in the field of hypertension and gut microbiota research, and to provide insights into the potential value of TCM in regulating the gut microbiota to prevent and treat hypertension. This study not only provides valuable academic references for researchers and medical practitioners, but also provides a practical scientific basis for public health policy makers and the public.
Different Gut Microbiota and Hypertension
The gut microbiota contains a high proportion of bacteria from Bacteroidetes, Firmicutes, Actinobacteria, and Proteobacteria.12 Table 1 summarises existing studies of different gut microbiota phyla in hypertension or individuals with higher BP, including study type, study subjects, sequencing methods, and variation in gut microbiota phyla.
![]() |
Table 1 Studies on Gut Microbiota Composition in Hypertension |
Gut Microbiota Imbalance
The uniformity, abundance, and diversity of the microbial community in the intestine are critical indicators reflecting the imbalance of the intestinal microbiota. In a healthy intestinal ecosystem, Bacteroidetes and Firmicutes are the dominant bacterial groups, and thus, the Firmicutes/Bacteroidetes (F/B) ratio can be regarded as a potential biomarker for pathological states.13 A study based on high-throughput sequencing technology using fecal samples from patients with grade 3 hypertension and healthy controls revealed that the gut microbiota of hypertensive patients exhibited significant decreases in abundance, diversity, and uniformity, with a notable increase in the F/B ratio. This increase was accompanied by an elevation in the abundance of Prevotella_9, Megasphaera, Parasutterella, and Escherichia Shigella, while the abundance of Bacteroides and Faecalibacterium decreased.14 The significant increase in the F/B ratio has been further validated in animal model studies.15,29 However, in cross-bred spontaneously hypertensive rat (SHR), this difference became particularly prominent, suggesting that relying solely on the F/B ratio as a predictor of disease status may have limitations.30 Therefore, a comprehensive analysis of the gut microbiota is crucial for accurately assessing pathological states such as hypertension.
Recent studies have shown that the gut microbiota can be regulated and balanced through diet and TCM, thereby increasing the numbers of Akkermansia, Bifidobacterium, and Bacteroides, and reducing the F/B ratio, which can help lower BP.31,32 In addition, oral administration of butyrate or acetate has been observed to prevent increases in BP and the F/B ratio in animal models.33 These results indicate that hypertension is associated with an unhealthy gut microbiota, and improving the gut microbiota may be a future target for the treatment of hypertension. Moreover, increases in the F/B ratio have also been observed in obesity and diabetes.34,35
Bacteroidetes
Bacteroidetes play an important role in protein metabolism in the gut and are strongly implicated in the development of hypertension. Most Bacteroidetes are gram-negative bacilli. The genera Bacteroides and Prevotella form a major part of the phylum Bacteroidetes, and their activities have a significant role in the decomposition and transformation of food.36 Bacteroides are the most abundant genus of bacteria in the gut and are thought to be a major source of short-chain fatty acids (SCFA) and an important contributor to the metabolism of dietary fibre and host-derived polysaccharides.37 Additionally, Prevotella contributes to the utilisation of plant materials in the rumen.38 Prevotella is found in the mouth, vagina, and intestine and can mediate intestinal mucosal inflammation, improve intestinal permeability and promote systemic inflammation.39,40 Many studies have found a significant increase in the presence of Prevotella in the stool of hypertensive patients.16–18 Both Bacteroides and Prevotella play an essential function in protein metabolism and possibly also in the metabolism of carcinogens and xenobiotics.
Firmicutes
There is a wide range of genera of bacteria in Firmicutes that have been strongly associated with hypertension. The majority of Firmicutes are gram-positive bacteria, including the Ruminococcus, Clostridium, and Veillonellaceae families. The abundance of Clostridium perfringens 1, Romboutsia, Ruminococcus 2, and Intestinibacter was found to be negatively correlated with SBP and diastolic blood pressure (DBP) in hypertensive patients receiving antihypertensive treatment.19 In addition, a decrease in the relative abundance of Ruminococcus was observed in the gut following a high-fat diet; Ruminococcus is the main producer of butyrate in the body.41 Butyrate is an SCFA that plays an active role in maintaining the overall health of the gut.42 Furthermore, in Dal salt-sensitive rats, the abundance of Veillonellaceae was positively correlated with BP,20 whereas Enterococci were more common in normotensive individuals.21
Proteobacteria
Proteobacteria have various shapes, are mainly defined by ribosomal ribonucleic acid (RNA) sequences and are commonly disorganised in the gut of hypertensive people. Proteobacteria are gram-negative bacteria, including species such as Klebsiella and Salmonella. The guts of patients with hypertension exhibit more Klebsiella and Salmonella bacteria than those of normotensive people.22 Klebsiella is a pathogen commonly found in the human intestinal tract and can cause a variety of infectious diseases, such as pneumonia, diarrhoea, and urinary tract infections.43,44 Furthermore, Su et al found dysbiosis of the gut microbiota in the intestines of hypertensive patients treated with antibiotics, resulting in large numbers of pathogenic gram-negative bacteria, such as Salmonella.23
Actinobacteria
The key genera of the Actinobacteria phylum, including Bifidobacterium and Mycobacterium, are both Gram-positive bacteria and crucial for the gut health of hypertensive patients. Bifidobacterium plays a significant role in reducing the production of lipopolysaccharide (LPS), maintaining the balance of the gut microbiota, and preserving the intestinal barrier function.45 A meta-analysis targeting the Chinese population has indicated that hypertensive patients suffer from impaired gut microbial diversity, manifesting as an increased F/B ratio, increased abundances of phylum Firmicutes, genus Megasphaera, Escherichia_Shigella, Klebsiella, and decreased abundances of Bacteroidaceae, Bifidobacterium, Faecalibacterium, Roseburia, and Ruminococcus.13 Additionally, a notable decrease in Bifidobacterium abundance has been observed in Ruminococcus-T1DM (type 1 diabetes mellitus) patients with high BP.24 This reduction in Bifidobacterium abundance is closely associated with an increased susceptibility to Mycobacterium tuberculosis among hypertensive patients. Therefore, Mycobacterium tuberculosis infection may serve as a common risk factor for both hypertension and tuberculosis.46,47
Metabolites of the Gut Microbiota
Short-Chain Fatty Acids
SCFA, as important metabolites of the gut microbiota, play a crucial role in the prevention of CVD such as hypertension.32,48–51 Studies have revealed that gut microbiota that produce SCFA are associated with reduced BP, while elevated concentrations of SCFA in feces are related to increased BP.52 In particular, butyrate can inhibit the production of proinflammatory cytokines, exert an anti-inflammatory effect, and affect BP by regulating the gut microbiota and the renin-angiotensin system.53–57 In recent years, there have been numerous reports on the use of specific probiotics or foods to improve butyrate-producing bacteria, helping to reduce the F/B ratio and BP in subjects.58–60 Additionally, SCFA receptors such as G-protein coupled receptors (mainly GPR41 and GPR43) and olfactory receptor 78 (Olfr78) play important roles in BP regulation.61,62 SCFA regulate the gut microbiota and affect BP levels by influencing these receptors and exerting anti-inflammatory effects.62–65 Therefore, optimizing the gut microbiota, especially by increasing the production of SCFA, may provide new strategies for the prevention and treatment of CVD such as hypertension (Figure 1).
![]() |
Figure 1 Effect of metabolites of gut microbiota on hypertension. Abbreviations: SCFA, short-chain fatty acid; TMA, trimethylamine; FMO3, flavin-containing monooxygenase 3; TMAO, trimethylamine N-oxide; LPS, lipopolysaccharide; Gpr41, G-protein coupled receptor 41; Olfr78, olfactory receptor 78. Notes: In Figure 1, the SCFA pathway, the TMAO pathway, and the LPS pathway are indicated by different coloured arrows, respectively. Created with BioRender.com. |
Trimethylamine N-Oxide
The concentration of trimethylamine N-oxide (TMAO) in the intestine is closely related to the BP of hypertensive patients, and its production is associated with the composition of the gut microbiota, diet, and disturbance of the intestinal blood barrier (Figure 1). TMAO is mainly generated by the oxidation of trimethylamine (TMA) by the gut microbiota, and its metabolic pathway differs in different regions of the intestine.66–69 Although the underlying mechanism between TMAO and hypertension is not fully understood, it has been confirmed that TMAO in the plasma can accelerate the formation of foam cells, enhance oxidative stress and proinflammatory responses, and reduce the production of anti-inflammatory cytokines.70 In hypertensive patients, due to the decreased intestinal barrier function, the inflammation in the body also increases when the concentration of TMAO rises.71,72 Multiple studies have shown that the level of plasma TMAO is associated with the risk of hypertension and other diseases, and there is a dose-dependent relationship.73–77 Animal models have demonstrated that specific intestinal microbiota are highly positively correlated with plasma TMA, TMAO levels, and the degree of atherosclerotic lesions, while certain bacterial flora show a negative correlation.78 Therefore, the production of TMAO may be influenced by individual differences in the gut microbiota, as indicated by the relationship between the F/B ratio and the degree of response to dietary precursors of TMAO.79
Lipopolysaccharide
LPS, commonly known as endotoxin, is a component of the bacterial wall of gram-negative bacteria and a key factor in triggering inflammation.80,81 In hypertensive patients with gut microbiota imbalance, the proliferation of gram-negative bacteria leads to an increase in LPS concentration, which then binds to Toll-like receptor 4 (TLR4) to activate the innate immune response. LPS forms a complex with LPS-binding protein (LBP), enters the blood from the intestine, interacts with CD14 on monocytes, and activates TLR4, thereby triggering the production of proinflammatory cytokines such as Tumour necrosis factor-α (TNF-α), interleukin-1 (IL-1), and IL-6.81,82 Butyrate, on the other hand, can inhibit the inflammatory response triggered by LPS.83 Studies have shown that prenatal exposure to LPS can induce oxidative stress, impair the ability to eliminate salt load, and lead to hypertension in multiple generations.84 Additionally, low-dose aspirin can reduce LPS-induced hypertension and related inflammatory responses in mice.85 Therefore, the systemic effects of LPS are closely related to the pathophysiology of hypertension (Figure 1).
Interaction Between Sympathetic Activation and the Gut-Brain
The imbalance of the gut microbiota exacerbates the progression of hypertension by activating the sympathetic drive and promoting neuroinflammation (as shown in Figure 2). Overactive sympathetic activity can directly or indirectly promote the development of hypertension and organ damage by stimulating low-grade systemic inflammation. Dysfunction of the gut-brain axis has been observed in animal models of hypertension.86 Additionally, increased sympathetic activation has been observed in the early stages of hypertension.87,88 Experiments involving FMT have confirmed the stimulatory effect of the gut microbiota on the sympathetic drive.89–91 Simultaneously, reducing sympathetic activity is crucial for alleviating structural changes in the cardiovascular system and mitigating hypertension.92,93
Different Hypertension Concomitant Factors
As previously stated, hypertension is caused by a variety of complex environmental factors. At present, the impact of exercise and diet on hypertension has attracted significant attention (Figure 3). Tables 2 and 3 summarise the interactions between exercise and diet and hypertension or higher BP, including study type, study population, intervention type, sequencing method, changes in gut microbiota and postintervention impact.
![]() |
Table 2 The Effect of Exercise on Gut Microbes |
![]() |
Table 3 The Effect of Diet on Gut Microbes |
![]() |
Figure 3 Effects of exercise, dietary pattern, and treatment on hypertension. Abbreviations: MD, Mediterranean diet; DASH, Dietary Approaches to Stop Hypertension; FMT, fecal microbiota transplantation; WD, Western diet. Notes: In Figure 3, the anti-hypertensive pathway and the pathway leading to hypertension are indicated by blue and red arrows, respectively. Created with BioRender.com. |
Exercise Changes Gut Microbiota
Exercise, as a non-pharmacological intervention, has shown significant results in the treatment and prevention of hypertension. Several studies have focused on athlete populations to reveal positive associations between their healthy BP status and the diversity of their gut microbiota, particularly the association between Prevotella counts and exercise duration and intensity.25,26 Although the extreme dietary and behavioural patterns of elite athletes limit the generalizability of the findings, studies in the general population have similarly shown that a combination of aerobic and resistance training can be effective in lowering BP and promoting gut microbiota diversity, with an increase in metabolites of beneficial microbes, such as short-chain fatty acids. These metabolites have biological activities such as anti-inflammatory and antioxidant properties that can directly or indirectly influence BP regulatory mechanisms.27 In addition, forms of exercise through harmonising the breath have shown significant positive effects in the treatment of hypertension. Among them, Liuzijue training (LZJ), a traditional exercise therapy that integrates breathing meditation with physical activity, has unique advantages in the prevention and improvement of hypertension. Compared with aerobic training, LZJ can effectively reduce BP, regulate immune homeostasis, and optimise the composition of the gut microbiota, and it is more easily applicable to the general population.28 In addition, yoga, Pilates, and traditional Chinese exercises (Tai Chi, Baduanjin, and Wuqinxi) are all complementary interventions for hypertension that focus on soothing exercise modalities that harmonise the breath, enhance flexibility, and promote inner peace and focus. It has been shown in many trials to help with BP control are safe and effective for hypertensive patients, and can be used as one of the means of rehabilitation.105–108 However, the role of these exercises in improving the gut microbiota still needs to be further explored in depth.
Although limited by sample size and confounding factors, the current study needs to further validate the beneficial effects of exercise on the gut microbiota in a large population. Given the specific physiological status of hypertensive patients, such as sympathetic hyperexcitability and exercise-induced BP elevation, exercise regimens should be more carefully tailored and individualised.109–111 In conclusion, despite the limitations of the study, the potential of exercise as a non-pharmacological therapy for hypertension has been preliminarily established, and future studies should further explore its specific applications and mechanisms in different populations.
Diet Regulates Blood Pressure and Gut Microbiota
There is a close relationship between diet and gut microbiota, inflammation, and BP levels.94–96 High salt/fat intake and changes in the composition and function of gut microbiota can lead to cardio-metabolic diseases, including hypertension, obesity, T2DM, and dyslipidemia.112 Among them, the Western diet (WD) contains little fiber, vitamins, minerals, or other plant-derived compounds (such as antioxidants), but is rich in refined sugar, salt, white flour, processed meat, animal fat, and food additives.113 This diet, rich in high salt, high fat, and processed foods, leads to gut microbiota imbalance, triggers inflammation, and increases the risk of hypertension.97,98 Experiments have shown that a high-salt diet reduces the abundance of Lactobacillus and the level of butyrate (a protective SCFA) in the intestines of mice, inducing a pro-inflammatory state.114,115 On the other hand, the Mediterranean diet (MD) and Dietary Approaches to Stop Hypertension (DASH) diet, characterized by low fat and high fiber, can effectively lower BP and improve cardiovascular health.99–101 Studies have shown that these dietary patterns increase gut microbial diversity, promote the growth of beneficial bacteria, reduce inflammatory markers, and effectively improve cardiovascular health.102–104,116 In summary, optimizing dietary patterns, especially reducing the intake of high-salt and high-fat foods and increasing the consumption of fiber-rich foods, is crucial for maintaining the balance of gut microbiota, reducing inflammation, and preventing hypertension.
Microbial Targeted Therapy
Probiotics
Probiotics have positive effects, including controlling intestinal flora, inhibiting inflammation, and boosting epithelial defence. This includes microorganisms that can produce SCFA, such as bifidobacteria, enterococci, and lactobacilli.117,118 Probiotics improve the structural and functional integrity of the gut wall and guard against neuroinflammation within the cardiac regulatory nucleus, which help to reduce hypertension. Probiotic (Kefir) intervention studies revealed higher serum LPS concentrations in SHR than in WKY rats and differences in the jejunal wall. The authors also reported decreased IL-6 and TNF-α protein density and abrogation of the activation of microglia in the hypothalamic paraventricular nucleus and medulla oblongata ventrolateral.119 A meta-analysis revealed that probiotic supplementation resulted in modest but significant reductions in SBP and DBP in hypertensive patients, an effect that was related to treatment duration, dose, and age of the subjects but not to the use of single or multiple strains. In addition, probiotic supplementation had beneficial effects on lowering BMI and blood glucose.120 Moreover, the beneficial effect of probiotics may be transmitted to offspring. In a rat trial of maternal flora-targeted therapy, probiotic or prebiotic therapy not only prevented elevated BP due to a perinatal high-fat diet and elevated BP in adult male offspring but also reduced maternal F/B, plasma TMAO levels, and TMAO to TMA ratios, as well as faecal concentrations of propionate and acetate in offspring, and increased the abundance of the genera Lactobacillus and Akkermansia.121 In summary, probiotics contribute to lowering BP by improving gut health and reducing inflammation, and these findings reveal the potential value of probiotics as a non-pharmacological treatment for the prevention and treatment of hypertension.
Faecal Microbiota Transplantation
The gut microbiota plays a critical role in the onset and progression of hypertension. FMT, which has attracted much interest in recent years, has been shown to not only directly alter the diversity of the gut flora and the proportion of cells that restore health but also to have fewer side effects and lower rates of recurrence and reinfection.122 According to a recent study, there was a significant decrease in SBP after 2 weeks of FMT from losartan-treated SHR to untreated SHR.123 Additionally, resveratrol can improve glucose imbalance and hypertension by affecting the gut flora.124 Researchers transplanted the gut microbiota of resveratrol-fed healthy mice into obese mice and improved glucose homeostasis in obese mice within 11 days. Hypertensive mice exhibited lower SBP within two weeks of FMT. Moreover, FMT was more effective than oral resveratrol for the same duration.125
However, individual differences are large, and the best FMT method, including donor screening and preparation, has not been determined.125,126 Patients with a history of resting ulcerative disease develop ulcerative colitis after FMT, which may be because the new gut microbiota triggers an immune response.127 This suggests that there are differences in the interactions of gut microbiota between individuals. Furthermore, the gut microbiota is hereditary and has been connected to several diseases (such as diabetes and colorectal cancer), with a theoretical risk of inadvertently promoting the development of these diseases.128,129 Moreover, the long-term impact of FMT is unclear, as most studies have been followed up for less than one year, and the hypothetical long-term adverse consequences of potentially harmful bacterial metastasis are unclear. As a result, to employ FMT effectively, a deeper understanding of the link between hypertension and gut flora in various individuals is needed.
Traditional Chinese Medicine
TCM has demonstrated remarkable efficacy in the clinical treatment of hypertension. Primarily through oral intervention, it effectively prevents and controls hypertension by regulating the structure and proportion of intestinal microbiota, thereby optimizing the immune function and metabolic mechanism of the body.130,131 Research has shown that TCM plays a crucial role in regulating and balancing intestinal flora. It can increase the number of beneficial bacteria such as Akkermansia, Bifidobacterium, and Bacteroides, and reduce the F/B ratio, creating a healthy environment for the intestine.30 For instance, Huanglian Jiedu Decoction (HJD) effectively prevents hypertension by increasing the diversity of SHR microbiota, reducing the frequency of S. solidus and enriching the abundance of lactobacilli spp.132 Moreover, it is noteworthy that HJD can significantly reduce the levels of inflammatory factors such as TNF-α and IL-8, effectively suppressing immune and inflammatory responses.133,134 Simultaneously, it significantly alters the gene expression profile of SHR, involving multiple cellular functions like smooth muscle contraction regulation, endothelial cell Ca(2+) balance, and nitric oxide (NO) pathways.135 In addition to HJD, the active components of Taohong Siwu Decoction (TSD) also demonstrate remarkable effects in the treatment of cardiovascular diseases. According to a meta-analysis, the combination of TSD and antihypertensive drugs can significantly enhance the antihypertensive effect, providing better treatment outcomes for hypertensive patients.136 The BuYang HuanWu decoction not only significantly enhances the gene expression of Bifidobacterium in the gut, but also reduces the gene expression of Escherichia coli, Clostridium, and Enterococcus. Moreover, it can significantly lower serum TMAO levels.137
It is worth mentioning that TCM can not only improve hypertension in combination with Western medicine but also alone, with effects superior to the use of Western medicine alone. Cheqianzi Cuduotang capsules can assist or be used in combination with Western medicine to improve hypertension symptoms by improving the intestinal microecology of elderly hypertensive patients, and its effect is even better than using Western medicine alone.138 In animal experiments, Eucommia ulmoides-Tribulus terrestris herbs have a positive impact on BP and inflammatory levels in elderly SHR, outperforming valsartan in maintaining the diversity of intestinal microbiota. Their antihypertensive mechanism is related to changes in the composition and diversity of intestinal microbiota.139 Additionally, Quanduzhong capsules, which contain extracts of Eucommia globulus, extract are very effective for patients with Grade 1 hypertension with low to moderate risk, reducing office SBP, DBP, and the 24-hour average coefficient of variation of DBP determined by 24-hour ambulatory BP monitoring.140
Compared with Western medicine, TCM formulas have the unique advantage of attacking multiple targets simultaneously. Zhengganxifeng decoction (ZGXFD) has been widely used in the clinical treatment of hypertension. Studies have shown that ZGXFD can significantly improve the structure of intestinal microbiota, reduce the F/B ratio and the ratio of cocci to bacilli (C/B), while maintaining the integrity of the intestinal mechanical barrier and increasing the proportion of bacteria that produce SCFA, providing a new strategy for hypertension treatment.141 Moreover, Zhijing powder can also regulate the signaling pathway of hypertension receptors, improve oxidative stress, and lower BP in rats with hypertension induced by cold stimulation and high salt intake.142
Regarding TCM decoctions for lowering BP, which include but are not limited to the examples mentioned above, their inclusion of a variety of medicinal materials allows for a combination effect of numerous bioactive components and pharmacological actions. This enables TCM decoctions to modulate the human body through multiple targets and pathways, thereby more comprehensively regulating physiological functions and achieving better antihypertensive effects. Moreover, there is literature suggesting that single TCMs may reduce the number of Bifidobacterium pseudolongum.143 Therefore, from the perspective of species diversity, TCM decoctions have better health benefits than single TCM preparations.
In summary, TCM has demonstrated remarkable efficacy and potential in the clinical treatment of hypertension (Figure 3). Compared with probiotics and FMT, TCM comprehensively regulates the body through various approaches and possesses more human experimental evidence and therapeutic effects, thus often becoming the preferred option for chronic disease treatment. It not only improves the symptoms of hypertensive patients but also enhances their quality of life, paving a new way for the treatment of hypertension.
Perspectives
The bacteria in the gut can create and secrete a wide range of compounds, allowing the gut microbiota to modulate hypertension in a variety of ways, frequently in collaboration. Therefore, a variety of therapies, such as dietary modification, exercise, probiotics, TCM and FMT, are being or will be used in the clinical setting to mitigate the development of hypertension (see Figure 3 for developmental pathways). However, due to heterogeneity, some therapies remain to be investigated in the general population. For example, when considering the gut microflora of professional athletes and the general population, it would be unrealistic to expect the general population to train in the same way as professional athletes and thus achieve a similar improvement in their gut microbiota. Moreover, the effects of probiotics vary depending on the disease and strain, and a protocol to lower BP using probiotics can only be achieved using specific probiotics. Moreover, although the results of animal experiments using probiotics have been satisfactory, human evidence is sparse and equivocal, and the function of certain strains is often unclear. As a result, probiotics are currently used for the primary prevention of hypertension and as a supplement to established medications for hypertension and do not reach the therapeutic threshold. In conclusion, diet, exercise and TCM may be effective ways to improve the gut microbiota in the long term, whereas the effectiveness of probiotics and FMT to improve the gut microbiota remains to be validated in large populations.
Conclusions
In recent years, there has been significant discussion regarding the relationship between the composition of the gut microbiota and the development of hypertension. The homogeneity of the distribution of microbiota in the gut, as well as the abundance and diversity in the gut, are important indicators of dysbiosis of the intestinal microbiota. For example, a decrease in mimetic bacteria and an increase in F/B can directly or indirectly contribute to a variety of diseases, including hypertension. Although the relationship between gut microbiota composition and elevated BP has not been thoroughly established, patients with an imbalanced gut microbiota are at higher risk for hypertension. Therefore, improving the gut microbiota may be a target for future hypertension treatment. Because most of our knowledge of these mechanisms comes from studies in animals and because there may be differences between the gut microbiota of animals and humans, caution should be exercised in extrapolating the results of animal experiments to humans. In the future, translating the various influences involved in hypertension to humans will remain a challenge. To better target potential therapeutic interventions, future studies with more representative population cohorts are needed to demonstrate the strengths and weaknesses of gut flora structure and function.
Abbreviations
BP, blood pressure; FMT, faecal microbiota transplantation; CVD, Cardiovascular disease; SBP, Systolic blood pressure; T2DM, type 2 diabetes mellitus; TCM, traditional Chinese Medicine; F/B, Firmicutes/Bacteroidetes; SHR, Spontaneously hypertensive rat; SCFA, short-chain fatty acids; DBP, Diastolic blood pressure; RNA, Ribonucleic Acid; LPS, Lipopolysaccharide; T1DM, Type 1 diabetes mellitus; GPR, G-protein coupled receptors; Olfr78 olfactory receptor 78; TMAO, Trimethylamine N-oxide; TMA, trimethylamine; TLR4, Toll-like receptor 4; LBP, LPS-binding protein; TNF-α, Tumour necrosis factor-α; IL-1, interleukin-1; LZJ, Liuzijue training; WD, Western diet; MD, Mediterranean diet; DASH, Dietary approach to stop hypertension; HJD, Huanglian Jiedu Decoction; TSD, Taohong Siwu Decoction; ZGXFD, Zhengganxifeng decoction; C/B, the ratio of cocci to bacilli.
Acknowledgments
Thanks for the support and help from the teachers and students in the research group and the Open Project Fund of the Key Laboratory of Cardiovascular and Cerebrovascular Diseases of the Ministry of Education (XN201907). The graphical abstract was Created with BioRender.com.
Author Contributions
From the initial concept and content framework design to the collection and collation of literature content necessary for the review, as well as the visual presentation of the article’s content, all authors have made significant contributions to the reported research. They were involved in the review and editing of the manuscript, approved the final publication draft, consented to submitting it to the selected journal, and agreed to take responsibility for all aspects of the work. Furthermore, all authors have reviewed and concurred with the published version of the manuscript.
Disclosure
The authors report no conflicts of interest in this work.
References
1. Saiz LC, Gorricho J, Garjón J, Celaya MC, Erviti J, Leache L. Blood pressure targets for the treatment of people with hypertension and cardiovascular disease. Cochrane Database Syst Rev. 2022;11(11):CD010315. doi:10.1002/14651858.CD010315.pub5
2. GBD 2019 Diseases and Injuries Collaborators. Global burden of 369 diseases and injuries in 204 countries and territories, 1990–2019: a systematic analysis for the Global Burden of Disease Study 2019. Lancet. 2020;396(10258):1204–1222. doi:10.1016/S0140-6736(20)30925-9
3. GBD 2019 Risk Factors Collaborators. Global burden of 87 risk factors in 204 countries and territories, 1990–2019: a systematic analysis for the Global Burden of Disease Study 2019. Lancet. 2020;396(10258):1223–1249. doi:10.1016/S0140-6736(20)30752-2
4. Kawarazaki W, Fujita T. Kidney and epigenetic mechanisms of salt-sensitive hypertension. Nat Rev Nephrol. 2021;17(5):350–363. doi:10.1038/s41581-021-00399-2
5. Tang WHW, Bäckhed F, Landmesser U, et al. Intestinal microbiota in cardiovascular health and disease: JACC State-of-The-Art Review. J Am Coll Cardiol. 2019;73(16):2089–2105. doi:10.1016/j.jacc.2019.03.024
6. Verhaar BJH, Prodan A, Nieuwdorp M, et al. Gut microbiota in hypertension and atherosclerosis: a review. Nutrients. 2020;12(10):2982. doi:10.3390/nu12102982
7. Duttaroy AK. Role of gut microbiota and their metabolites on atherosclerosis, hypertension and human blood platelet function: a review. Nutrients. 2021;13(1):144. doi:10.3390/nu13010144
8. Joe B, McCarthy CG, Edwards JM, et al. Microbiota introduced to germ-free rats restores vascular contractility and blood pressure. Hypertension. 2020;76(6):1847–1855. doi:10.1161/HYPERTENSIONAHA.120.15939
9. Tindall AM, McLimans CJ, Petersen KS, et al. Walnuts and vegetable oils containing oleic acid differentially affect the gut microbiota and associations with cardiovascular risk factors: follow-up of a randomized, controlled, feeding trial in adults at risk for cardiovascular disease. J Nutr. 2020;150(4):806–817. doi:10.1093/jn/nxz289
10. Koeth RA, Lam-Galvez BR, Kirsop J, et al. l-Carnitine in omnivorous diets induces an atherogenic gut microbial pathway in humans. J Clin Invest. 2019;129(1):373–387. doi:10.1172/JCI94601
11. Liu B, Zhang L, Yang H, et al. Microbiota: a potential orchestrator of antidiabetic therapy. Front Endocrinol. 2023;14:973624. doi:10.3389/fendo.2023.973624
12. Qin J, Li R, Raes J, et al. A human gut microbial gene catalogue established by metagenomic sequencing. Nature. 2010;464(7285):59–65. doi:10.1038/nature08821
13. Qin Y, Zhao J, Wang Y, et al. Specific alterations of gut microbiota in Chinese patients with hypertension: a systematic review and meta-analysis. Kidney Blood Press Res. 2022;47(7):433–447. doi:10.1159/000524282
14. Mushtaq N, Hussain S, Zhang S, et al. Molecular characterization of alterations in the intestinal microbiota of patients with grade 3 hypertension. Int J Mol Med. 2019;44(2):513–522. doi:10.3892/ijmm.2019.4235
15. Yan X, Jin J, Su X, et al. Intestinal flora modulates blood pressure by regulating the synthesis of intestinal-derived corticosterone in high salt-induced hypertension. Circ Res. 2020;126(7):839–853. doi:10.1161/CIRCRESAHA.119.316394
16. Dinakis E, Nakai M, Gill P, et al. Association between the gut microbiome and their metabolites with human blood pressure variability. Hypertension. 2022;79(8):1690–1701. doi:10.1161/HYPERTENSIONAHA.122.19350
17. Han ZL, Chen M, Fu XD, et al. Potassium alginate oligosaccharides alter gut microbiota, and have potential to prevent the development of hypertension and heart failure in spontaneously hypertensive rats. Int J Mol Sci. 2021;22(18):9823. doi:10.3390/ijms22189823
18. Fang C, Zuo K, Fu Y, et al. Aggravated gut microbiota and metabolomic imbalances are associated with hypertension patients comorbid with atrial fibrillation. Biomolecules. 2022;12(10):1445. doi:10.3390/biom12101445
19. Li H, Liu B, Song J, et al. Characteristics of gut microbiota in patients with hypertension and/or hyperlipidemia: a cross-sectional study on rural residents in Xinxiang County, Henan Province. Microorganisms. 2019;7(10):399. doi:10.3390/microorganisms7100399
20. Galla S, Chakraborty S, Cheng X, et al. Exposure to amoxicillin in early life is associated with changes in gut microbiota and reduction in blood pressure: findings from a study on rat dams and offspring. J Am Heart Assoc. 2020;9(2):e014373. doi:10.1161/JAHA.119.014373
21. Dan X, Mushi Z, Baili W, et al. Differential analysis of hypertension-associated intestinal microbiota. Int J Med Sci. 2019;16(6):872–881. doi:10.7150/ijms.29322
22. Yan Q, Gu Y, Li X, et al. Alterations of the Gut Microbiome in Hypertension. Front Cell Infect Microbiol. 2017;7:381. doi:10.3389/fcimb.2017.00381
23. Su C, Liu Y, Zhang H, et al. Investigation of the effects of antibiotic application on the intestinal flora in elderly hypertension patients with infectious diseases. Iran J Public Health. 2018;47(3):335–341.
24. Lakshmanan AP, Shatat IF, Zaidan S, et al. Bifidobacterium reduction is associated with high blood pressure in children with type 1 diabetes mellitus. Biomed Pharmacother. 2021;140:111736. doi:10.1016/j.biopha.2021.111736
25. Barton W, Penney NC, Cronin O, et al. The microbiome of professional athletes differs from that of more sedentary subjects in composition and particularly at the functional metabolic level. Gut. 2018;67(4):625–633. doi:10.1136/gutjnl-2016-313627
26. Kulecka M, Fraczek B, Mikula M, et al. The composition and richness of the gut microbiota differentiate the top Polish endurance athletes from sedentary controls. Gut Microbes. 2020;11(5):1374–1384. doi:10.1080/19490976.2020.1758009
27. Zhong F, Xu Y, Lai HY, et al. Effects of combined aerobic and resistance training on gut microbiota and cardiovascular risk factors in physically active elderly women: a randomized controlled trial. Front Physiol. 2022;13:1004863. doi:10.3389/fphys.2022.1004863
28. Wu S, Zheng C, Liu N, et al. Liuzijue training improves hypertension and modulates gut microbiota profile. Front Cardiovasc Med. 2023;10:1075084. doi:10.3389/fcvm.2023.1075084
29. Abboud FM, Cicha MZ, Ericsson A, et al. Altering early life gut microbiota has long-term effect on immune system and hypertension in spontaneously hypertensive rats. Front Physiol. 2021;12:752924. doi:10.3389/fphys.2021.752924
30. Magne F, Gotteland M, Gauthier L, et al. The Firmicutes/bacteroidetes ratio: a relevant marker of gut dysbiosis in obese patients? Nutrients. 2020;12(5):1474. doi:10.3390/nu12051474
31. Zhang GX, Jin L, Jin H, et al. Influence of dietary components and traditional Chinese medicine on hypertension: a potential role for gut microbiota. Evid Based Complement Alternat Med. 2021;2021:5563073. doi:10.1155/2021/5563073
32. Daliri EB, Ofosu FK, Chelliah R, et al. Influence of fermented soy protein consumption on hypertension and gut microbial modulation in spontaneous hypertensive rats. Biosci Microbiota Food Health. 2020;39(4):199–208. doi:10.12938/bmfh.2020-001
33. Wang Q, He Y, Li X, et al. Lactobacillus reuteri CCFM8631 alleviates hypercholesterolaemia caused by the paigen atherogenic diet by regulating the gut microbiota. Nutrients. 2022;14(6):1272. doi:10.3390/nu14061272
34. Cheng L, Chen Y, Zhang X, et al. A metagenomic analysis of the modulatory effect of Cyclocarya paliurus flavonoids on the intestinal microbiome in a high-fat diet-induced obesity mouse model. J Sci Food Agric. 2019;99(8):3967–3975. doi:10.1002/jsfa.9622
35. Qiu J, Zhou H, Jing Y, et al. Association between blood microbiome and type 2 diabetes mellitus: a nested case-control study. J Clin Lab Anal. 2019;33(4):e22842. doi:10.1002/jcla.22842
36. Veloo ACM, Baas WH, Haan FJ, et al. Prevalence of antimicrobial resistance genes in Bacteroides spp. and Prevotella spp. Dutch clinical isolates. Clin Microbiol Infect. 2019;25(9):1156.e9–1156.e13. doi:10.1016/j.cmi.2019.02.017
37. Ryan D, Prezza G, Westermann AJ. An RNA-centric view on gut Bacteroidetes. Biol Chem. 2020;402(1):55–72. doi:10.1515/hsz-2020-0230
38. Su M, Hao Z, Shi H, et al. Metagenomic analysis revealed differences in composition and function between liquid-associated and solid-associated microorganisms of sheep rumen. Front Microbiol. 2022;13:851567. doi:10.3389/fmicb.2022.851567
39. Pignatelli P, Romei FM, Bondi D, et al. Microbiota and oral cancer as a complex and dynamic microenvironment: a narrative review from etiology to prognosis. Int J Mol Sci. 2022;23(15):8323. doi:10.3390/ijms23158323
40. Gao J, Peng Y, Jiang N, et al. High-throughput sequencing-based analysis of changes in the vaginal microbiome during the disease course of patients with bacterial vaginosis: a case-control study. Biology. 2022;11(12):1797. doi:10.3390/biology11121797
41. Ding L, Liu J, Zhou L, et al. A high-fat diet disrupts the hepatic and adipose circadian rhythms and modulates the diurnal rhythm of gut microbiota-derived short-chain fatty acids in gestational mice. Front Nutr. 2022;9:925390. doi:10.3389/fnut.2022.925390
42. Li Z, Zhou E, Liu C, et al. Dietary butyrate ameliorates metabolic health associated with selective proliferation of gut Lachnospiraceae bacterium 28-4. JCI Insight. 2023;8(4):e166655. doi:10.1172/jci.insight.166655
43. Sheikh Omar NM, Erismis B, Muse Osman M, et al. Retrospective evaluation of nosocomial bacterial infections and their antimicrobial resistance patterns among hospitalized patients in Mogadishu, Somalia. Infect Drug Resist. 2023;16:705–720. doi:10.2147/IDR.S398387
44. Rojas R, Macesic N, Tolari G, et al. Multidrug-Resistant Klebsiella pneumoniae ST307 in traveler returning from Puerto Rico to Dominican Republic. Emerg Infect Dis. 2019;25(8):1583–1585. doi:10.3201/eid2508.171730
45. Yue Y, Wang Y, Xie Q, et al. Bifidobacterium bifidum E3 Combined with Bifidobacterium longum subsp. infantis E4 Improves LPS-Induced Intestinal Injury by Inhibiting the TLR4/NF-κB and MAPK Signaling Pathways In Vivo. J Agric Food Chem. 2023;71(23):8915–8930. doi:10.1021/acs.jafc.3c00421
46. Chidambaram V, Gupte A, Wang JY, et al. The impact of hypertension and use of calcium channel blockers on tuberculosis treatment outcomes. Clin Infect Dis. 2021;73(9):e3409–e3418. doi:10.1093/cid/ciaa1446
47. Guo S, Lei S, Palittapongarnpim P, et al. Association between Mycobacterium tuberculosis genotype and diabetes mellitus/hypertension: a molecular study. BMC Infect Dis. 2022;22(1):401. doi:10.1186/s12879-022-07344-z
48. Morrison DJ, Preston T. Formation of short chain fatty acids by the gut microbiota and their impact on human metabolism. Gut Microbes. 2016;7(3):189–200. doi:10.1080/19490976.2015.1134082
49. Li L, Zhong SJ, Hu SY, et al. Changes of gut microbiome composition and metabolites associated with hypertensive heart failure rats. BMC Microbiol. 2021;21(1):141. doi:10.1186/s12866-021-02202-5
50. Zuo K, Fang C, Liu Z, et al. Commensal microbe-derived SCFA alleviates atrial fibrillation via GPR43/NLRP3 signaling. Int J Biol Sci. 2022;18(10):4219–4232. doi:10.7150/ijbs.70644
51. Thananimit S, Pahumunto N, Teanpaisan R. Characterization of short chain fatty acids produced by selected potential probiotic lactobacillus strains. Biomolecules. 2022;12(12):1829. doi:10.3390/biom12121829
52. Sun S, Lulla A, Sioda M, et al. Gut microbiota composition and blood pressure. Hypertension. 2019;73(5):998–1006. doi:10.1161/HYPERTENSIONAHA.118.12109
53. Soto-Martin EC, Warnke I, Farquharson FM, et al. Vitamin biosynthesis by human gut butyrate-producing bacteria and cross-feeding in synthetic microbial communities. mBio. 2020;11(4):e00886–20. doi:10.1128/mBio.00886-20
54. Tilves C, Yeh HC, Maruthur N, et al. Increases in circulating and fecal butyrate are associated with reduced blood pressure and hypertension: results from the SPIRIT trial. J Am Heart Assoc. 2022;11(13):e024763. doi:10.1161/JAHA.121.024763
55. Li G, Tian Y, Zhu WG. The roles of histone deacetylases and their inhibitors in cancer therapy. Front Cell Dev Biol. 2020;8:576946. doi:10.3389/fcell.2020.576946
56. Makkar R, Behl T, Arora S. Role of HDAC inhibitors in diabetes mellitus. Curr Res Transl Med. 2020;68(2):45–50. doi:10.1016/j.retram.2019.08.001
57. Hsu CN, Yu HR, Lin IC, et al. Sodium butyrate modulates blood pressure and gut microbiota in maternal tryptophan-free diet-induced hypertension rat offspring. J Nutr Biochem. 2022;108:109090. doi:10.1016/j.jnutbio.2022.109090
58. Robles-Vera I, Toral M, de la Visitación N, et al. Probiotics prevent dysbiosis and the rise in blood pressure in genetic hypertension: role of short-chain fatty acids. Mol Nutr Food Res. 2020;64(6):e1900616. doi:10.1002/mnfr.201900616
59. Le Roy T, Moens de Hase E, Van Hul M, et al. Dysosmobacter welbionis is a newly isolated human commensal bacterium preventing diet-induced obesity and metabolic disorders in mice. Gut. 2022;71(3):534–543. doi:10.1136/gutjnl-2020-323778
60. Le Sayec M, Xu Y, Laiola M, et al. The effects of Aronia berry (poly)phenol supplementation on arterial function and the gut microbiome in middle aged men and women: results from a randomized controlled trial. Clin Nutr. 2022;41(11):2549–2561. doi:10.1016/j.clnu.2022.08.024
61. Ilyés T, Silaghi CN, Crăciun AM. Diet-related changes of short-chain fatty acids in blood and feces in obesity and metabolic syndrome. Biology. 2022;11(11):1556. doi:10.3390/biology11111556
62. Xu J, Moore BN, Pluznick JL. Short-chain fatty acid receptors and blood pressure regulation: Council on Hypertension Mid-Career Award for Research Excellence 2021. Hypertension. 2022;79(10):2127–2137. doi:10.1161/HYPERTENSIONAHA.122.18558
63. Dardi P, Dos Santos-Eichler RA, de Oliveira S, et al. Reduced intestinal butyrate availability is associated with the vascular remodeling in resistance arteries of hypertensive rats. Front Physiol. 2022;13:998362. doi:10.3389/fphys.2022.998362
64. Kaye DM, Shihata WA, Jama HA, et al. Deficiency of prebiotic fiber and insufficient signaling through gut metabolite-sensing receptors leads to cardiovascular disease. Circulation. 2020;141(17):1393–1403. doi:10.1161/CIRCULATIONAHA.119.043081
65. Hsu CN, Hou CY, Chang CI, et al. Resveratrol butyrate ester protects adenine-treated rats against hypertension and kidney disease by Regulating the Gut-Kidney Axis. Antioxidants. 2021;11(1):83. doi:10.3390/antiox11010083
66. Zeisel SH, Warrier M. Trimethylamine N-Oxide, the microbiome, and heart and kidney disease. Annu Rev Nutr. 2017;37:157–181. doi:10.1146/annurev-nutr-071816-064732
67. Koeth RA, Wang Z, Levison BS, et al. Intestinal microbiota metabolism of L-carnitine, a nutrient in red meat, promotes atherosclerosis. Nat Med. 2013;19(5):576–585. doi:10.1038/nm.3145
68. Maksymiuk KM, Szudzik M, Gawryś-Kopczyńska M, et al. Trimethylamine, a gut bacteria metabolite and air pollutant, increases blood pressure and markers of kidney damage including proteinuria and KIM-1 in rats. J Transl Med. 2022;20(1):470. doi:10.1186/s12967-022-03687-y
69. Romano KA, Vivas EI, Amador-Noguez D, et al. Intestinal microbiota composition modulates choline bioavailability from diet and accumulation of the proatherogenic metabolite trimethylamine-N-oxide. mBio. 2015;6(2):e02481. doi:10.1128/mBio.02481-14
70. Brunt VE, Greenberg NT, Sapinsley ZJ, et al. Suppression of trimethylamine N-oxide with DMB mitigates vascular dysfunction, exercise intolerance, and frailty associated with a Western-style diet in mice. J Appl Physiol. 2022;133(4):798–813. doi:10.1152/japplphysiol.00350.2022
71. Oh S, Shim M, Son M, et al. Attenuating effects of dieckol on endothelial cell dysfunction via modulation of Th17/Treg balance in the intestine and aorta of spontaneously hypertensive rats. Antioxidants. 2021;10(2):298. doi:10.3390/antiox10020298
72. Madhur MS, Elijovich F, Alexander MR, et al. Hypertension: do inflammation and immunity hold the key to solving this epidemic? Circ Res. 2021;128(7):908–933. doi:10.1161/CIRCRESAHA.121.318052
73. Wang Z, Klipfell E, Bennett BJ, et al. Gut flora metabolism of phosphatidylcholine promotes cardiovascular disease. Nature. 2011;472(7341):57–63. doi:10.1038/nature09922
74. Barrea L, Muscogiuri G, Annunziata G, et al. A new light on vitamin d in obesity: a novel association with Trimethylamine-N-Oxide (TMAO). Nutrients. 2019;11(6):1310. doi:10.3390/nu11061310
75. Al-Rubaye H, Perfetti G, Kaski JC. The role of microbiota in cardiovascular risk: focus on trimethylamine oxide. Curr Probl Cardiol. 2019;44(6):182–196. doi:10.1016/j.cpcardiol.2018.06.005
76. Krishnan S, O’Connor LE, Wang Y, et al. Adopting a Mediterranean-style eating pattern with low, but not moderate, unprocessed, lean red meat intake reduces fasting serum trimethylamine N-oxide (TMAO) in adults who are overweight or obese. Br J Nutr. 2022;128(9):1–21. doi:10.1017/S0007114521004694
77. Ge X, Zheng L, Zhuang R, et al. The gut microbial metabolite trimethylamine N-Oxide and hypertension risk: a systematic review and dose-response meta-analysis. Adv Nutr. 2020;11(1):66–76. doi:10.1093/advances/nmz064
78. Wang Z, Roberts AB, Buffa JA, et al. Non-lethal inhibition of gut microbial trimethylamine production for the treatment of atherosclerosis. Cell. 2015;163(7):1585–1595. doi:10.1016/j.cell.2015.11.055
79. Allaway D, Haydock R, Lonsdale ZN, et al. Rapid reconstitution of the fecal microbiome after extended diet-induced changes indicates a stable gut microbiome in healthy adult dogs. Appl Environ Microbiol. 2020;86(13):e00562–20. doi:10.1128/AEM.00562-20
80. Zhang HX, Li YY, Liu ZJ, et al. Quercetin effectively improves LPS-induced intestinal inflammation, pyroptosis, and disruption of the barrier function through the TLR4/NF-κB/NLRP3 signaling pathway in vivo and in vitro. Food Nutr Res. 2022;66:8948. doi:10.29219/fnr.v66.8948
81. Liu J, Chang G, Huang J, et al. Sodium butyrate inhibits the inflammation of lipopolysaccharide-induced acute lung injury in mice by regulating the Toll-Like Receptor 4/Nuclear Factor κB signaling pathway. J Agric Food Chem. 2019;67(6):1674–1682. doi:10.1021/acs.jafc.8b06359
82. Ciesielska A, Matyjek M, Kwiatkowska K. TLR4 and CD14 trafficking and its influence on LPS-induced pro-inflammatory signaling. Cell Mol Life Sci. 2021;78(4):1233–1261. doi:10.1007/s00018-020-03656-y
83. Wang H, Song W, Wu Q, et al. Fecal transplantation from db/db mice treated with sodium butyrate attenuates ischemic stroke injury. Microbiol Spectr. 2021;9(2):e00042–21. doi:10.1128/Spectrum.00042-21
84. Cao N, Lan C, Chen C, et al. Prenatal lipopolysaccharides exposure induces transgenerational inheritance of hypertension. Circulation. 2022;146(14):1082–1095. doi:10.1161/CIRCULATIONAHA.122.059891
85. Li G, Wei W, Suo L, et al. Low-dose aspirin prevents kidney damage in LPS-induced preeclampsia by inhibiting the WNT5A and NF-κB signaling pathways. Front Endocrinol. 2021;12:639592. doi:10.3389/fendo.2021.639592
86. Santisteban MM, Qi Y, Zubcevic J, et al. Hypertension-linked pathophysiological alterations in the gut. Circ Res. 2017;120(2):312–323. doi:10.1161/CIRCRESAHA.116.309006
87. Ye C, Zheng F, Wang JX, et al. Dysregulation of the excitatory renal reflex in the sympathetic activation of spontaneously hypertensive rat. Front Physiol. 2021;12:673950. doi:10.3389/fphys.2021.673950
88. Haburčák M, Harrison J, Buyukozturk MM, et al. Heightened sympathetic neuron activity and altered cardiomyocyte properties in spontaneously hypertensive rats during the postnatal period. Front Synaptic Neurosci. 2022;14:995474. doi:10.3389/fnsyn.2022.995474
89. Qi J, Fu LY, Liu KL, et al. Resveratrol in the hypothalamic paraventricular nucleus attenuates hypertension by regulation of ROS and neurotransmitters. Nutrients. 2022;14(19):4177. doi:10.3390/nu14194177
90. Gao HL, Yu XJ, Feng YQ, et al. Luteolin attenuates hypertension via inhibiting NF-κB-mediated inflammation and PI3K/Akt signaling pathway in the hypothalamic paraventricular nucleus. Nutrients. 2023;15(3):502. doi:10.3390/nu15030502
91. Xia WJ, Xu ML, Yu XJ, et al. Antihypertensive effects of exercise involve reshaping of gut microbiota and improvement of gut-brain axis in spontaneously hypertensive rat. Gut Microbes. 2021;13(1):1–24. doi:10.1080/19490976.2020.1854642
92. Pan Y, Sun S, Wang X, et al. Improvement of vascular function by knockdown of salusin-β in hypertensive rats via nitric oxide and reactive oxygen species signaling pathway. Front Physiol. 2021;12:622954. doi:10.3389/fphys.2021.622954
93. Sun S, Zhang F, Pan Y, et al. A TOR2A gene product: salusin-β contributes to attenuated vasodilatation of spontaneously hypertensive rats. Cardiovasc Drugs Ther. 2021;35(1):125–139. doi:10.1007/s10557-020-06983-1
94. David LA, Maurice CF, Carmody RN, et al. Diet rapidly and reproducibly alters the human gut microbiome. Nature. 2014;505(7484):559–563. doi:10.1038/nature12820
95. Wan Y, Yuan J, Li J, et al. Unconjugated and secondary bile acid profiles in response to higher-fat, lower-carbohydrate diet and associated with related gut microbiota: a 6-month randomized controlled-feeding trial. Clin Nutr. 2020;39(2):395–404. doi:10.1016/j.clnu.2019.02.037
96. Cho KY. Lifestyle modifications result in alterations in the gut microbiota in obese children. BMC Microbiol. 2021;21(1):10. doi:10.1186/s12866-020-02002-3
97. Andújar-Tenorio N, Prieto I, Cobo A, et al. High fat diets induce early changes in gut microbiota that may serve as markers of ulterior altered physiological and biochemical parameters related to metabolic syndrome. Effect of virgin olive oil in comparison to butter. PLoS One. 2022;17(8):e0271634. doi:10.1371/journal.pone.0271634
98. Vileigas DF, Marciano CLC, Mota GAF, et al. Temporal measures in cardiac structure and function during the development of obesity induced by different types of western diet in a rat model. Nutrients. 2019;12(1):68. doi:10.3390/nu12010068
99. Filippou CD, Tsioufis CP, Thomopoulos CG, et al. Dietary Approaches to Stop Hypertension (DASH) diet and blood pressure reduction in adults with and without hypertension: a systematic review and meta-analysis of randomized controlled trials. Adv Nutr. 2020;11(5):1150–1160. doi:10.1093/advances/nmaa041
100. Li J, Guasch-Ferré M, Chung W, et al. The Mediterranean diet, plasma metabolome, and cardiovascular disease risk. Eur Heart J. 2020;41(28):2645–2656. doi:10.1093/eurheartj/ehaa209
101. Schwingshackl L, Chaimani A, Schwedhelm C, et al. Comparative effects of different dietary approaches on blood pressure in hypertensive and pre-hypertensive patients: a systematic review and network meta-analysis. Crit Rev Food Sci Nutr. 2019;59(16):2674–2687. doi:10.1080/10408398.2018.1463967
102. Jennings A, Berendsen AM, de Groot GM, et al. Mediterranean-style diet improves systolic blood pressure and arterial stiffness in older adults. Hypertension. 2019;73(3):578–586. doi:10.1161/HYPERTENSIONAHA.118.12259
103. Nagpal R, Shively CA, Appt SA, et al. Gut microbiome composition in non-human primates consuming a western or Mediterranean diet. Front Nutr. 2018;5:28. doi:10.3389/fnut.2018.00028
104. Belanger MJ, Kovell LC, Turkson-Ocran RA, et al. Effects of the dietary approaches to stop hypertension diet on change in cardiac biomarkers over time: results from the DASH-sodium trial. J Am Heart Assoc. 2023;12(2):e026684. doi:10.1161/JAHA.122.026684
105. Cramer H, Sellin C, Schumann D, et al. Yoga in arterial hypertension. Dtsch Arztebl Int. 2018;115(50):833–839. doi:10.3238/arztebl.2018.0833
106. Nalbant G, Hassanein ZM, Lewis S, et al. Content, structure, and delivery characteristics of yoga interventions for managing hypertension: a systematic review and meta-analysis of randomized controlled trials. Front Public Health. 2022;10:846231. doi:10.3389/fpubh.2022.846231
107. Rocha J, Cunha FA, Cordeiro R, et al. Acute effect of a single session of pilates on blood pressure and cardiac autonomic control in middle-aged adults with hypertension. J Strength Cond Res. 2020;34(1):114–123. doi:10.1519/JSC.0000000000003060
108. Dai L, Jiang Y, Wang P, et al. Effects of three traditional Chinese fitness exercises combined with antihypertensive drugs on patients with essential hypertension: a systematic review and network meta-analysis of randomized controlled trials. Evid Based Complement Alternat Med. 2021;2021:2570472. doi:10.1155/2021/2570472
109. Iyonaga T, Shinohara K, Mastuura T, et al. Brain perivascular macrophages contribute to the development of hypertension in stroke-prone spontaneously hypertensive rats via sympathetic activation. Hypertens Res. 2020;43(2):99–110. doi:10.1038/s41440-019-0333-4
110. Syme C, Shin J, Richer L, et al. Sex differences in blood pressure hemodynamics in middle-aged adults with overweight and obesity. Hypertension. 2019;74(2):407–412. doi:10.1161/HYPERTENSIONAHA.119.13058
111. Matsukawa K, Iwamoto GA, Mitchell JH, et al. Exaggerated renal sympathetic nerve and pressor responses during spontaneously occurring motor activity in hypertensive rats. Am J Physiol Regul Integr Comp Physiol. 2023;324(4):R497–R512. doi:10.1152/ajpregu.00271.2022
112. DeMarco VG, Aroor AR, Sowers JR. The pathophysiology of hypertension in patients with obesity. Nat Rev Endocrinol. 2014;10(6):364–376. doi:10.1038/nrendo.2014.44
113. An J, Zhao X, Wang Y, et al. Western-style diet impedes colonization and clearance of Citrobacter rodentium. PLoS Pathog. 2021;17(4):e1009497. doi:10.1371/journal.ppat.1009497
114. Bier A, Braun T, Khasbab R, et al. A high salt diet modulates the gut microbiota and short chain fatty acids production in a salt-sensitive hypertension rat model. Nutrients. 2018;10(9):1154. doi:10.3390/nu10091154
115. Miranda PM, De Palma G, Serkis V, et al. High salt diet exacerbates colitis in mice by decreasing Lactobacillus levels and butyrate production. Microbiome. 2018;6(1):57. doi:10.1186/s40168-018-0433-4
116. Rebholz CM, Lichtenstein AH, Zheng Z, et al. Serum untargeted metabolomic profile of the Dietary Approaches to Stop Hypertension (DASH) dietary pattern. Am J Clin Nutr. 2018;108(2):243–255. doi:10.1093/ajcn/nqy099
117. Liu Q, Yu Z, Tian F, et al. Surface components and metabolites of probiotics for regulation of intestinal epithelial barrier. Microb Cell Fact. 2020;19(1):23. doi:10.1186/s12934-020-1289-4
118. Zhou L, Ding C, Wu J, et al. Probiotics and synbiotics show clinical efficacy in treating gestational diabetes mellitus: a meta-analysis. Prim Care Diabetes. 2021;15(6):937–947. doi:10.1016/j.pcd.2021.08.005
119. de Almeida Silva M, Mowry FE, Peaden SC, et al. Kefir ameliorates hypertension via gut-brain mechanisms in spontaneously hypertensive rats. J Nutr Biochem. 2020;77:108318. doi:10.1016/j.jnutbio.2019.108318
120. Chi C, Li C, Wu D, et al. Effects of probiotics on patients with hypertension: a systematic review and meta-analysis. Curr Hypertens Rep. 2020;22(5):34. doi:10.1007/s11906-020-01042-4
121. Hsu CN, Hou CY, Chan JYH, et al. Hypertension programmed by perinatal high-fat diet: effect of maternal gut microbiota-targeted therapy. Nutrients. 2019;11(12):2908. doi:10.3390/nu11122908
122. Fan L, Ren J, Chen Y, et al. Effect of fecal microbiota transplantation on primary hypertension and the underlying mechanism of gut microbiome restoration: protocol of a randomized, blinded, placebo-controlled study. Trials. 2022;23(1):178. doi:10.1186/s13063-022-06086-2
123. Robles-Vera I, Toral M, de la Visitación N, et al. Changes to the gut microbiota induced by losartan contributes to its antihypertensive effects. Br J Pharmacol. 2020;177(9):2006–2023. doi:10.1111/bph.14965
124. Kim TT, Parajuli N, Sung MM, et al. Fecal transplant from resveratrol-fed donors improves glycaemia and cardiovascular features of the metabolic syndrome in mice. Am J Physiol Endocrinol Metab. 2018;315(4):E511–E519. doi:10.1152/ajpendo.00471.2017
125. Lai CY, Sung J, Cheng F, et al. Systematic review with meta-analysis: review of donor features, procedures and outcomes in 168 clinical studies of faecal microbiota transplantation. Aliment Pharmacol Ther. 2019;49(4):354–363. doi:10.1111/apt.15116
126. Dawwas GK, Brensinger CM, Vajravelu RK, et al. Long-term outcomes following multiply recurrent clostridioides difficile infection and fecal microbiota transplantation. Clin Gastroenterol Hepatol. 2022;20(4):806–816.e6. doi:10.1016/j.cgh.2020.12.004
127. Lopetuso LR, Ianiro G, Allegretti JR, et al. Fecal transplantation for ulcerative colitis: current evidence and future applications. Expert Opin Biol Ther. 2020;20(4):343–351. doi:10.1080/14712598.2020.1733964
128. Cheng Y, Ling Z, Li L. The intestinal microbiota and colorectal cancer. Front Immunol. 2020;11:615056. doi:10.3389/fimmu.2020.615056
129. Alvarez-Silva C, Kashani A, Hansen TH, et al. Trans-ethnic gut microbiota signatures of type 2 diabetes in Denmark and India. Genome Med. 2021;13(1):37. doi:10.1186/s13073-021-00856-4
130. Xu YQ, Yuan JL, Niu HT. Progress in research on the prevention of hypertension by regulating intestinal flora with traditional Chinese medicine. Chin J Microecol. 2021;5:599–602+608. doi:10.13381/j.cnki.cjm.202105022
131. Yang M, Lao L. Emerging applications of metabolomics in traditional Chinese medicine treating hypertension: biomarkers, pathways and more. Front Pharmacol. 2019;10:158. doi:10.3389/fphar.2019.00158
132. Ma XC, Xiong XJ, Mo Y, et al. Study on changes of intestinal microflora in spontaneous sly hypertensive rats based on 16S rDNA sequencing and intervention of traditional Chinese Medicine. Chinese Archives Traditional Chin Med. 2020;8:71–74+265–266. doi:10.13193/j.issn.1673-7717.2020.08.017
133. Luo K, Zhao H, Bian B, et al. Huanglian Jiedu Decoction in the treatment of the Traditional Chinese Medicine Syndrome “Shanghuo”-an intervention study. Front Pharmacol. 2021;12:616318. doi:10.3389/fphar.2021.616318
134. Li X, Wei S, Ma X, et al. Huanglian Jiedu Decoction Exerts Antipyretic effect by inhibiting MAPK signaling pathway. Evid Based Complement Alternat Med. 2021;2021:2209574. doi:10.1155/2021/2209574
135. Yue GH, Zhuo SY, Xia M, et al. Effect of huanglian jiedu decoction on thoracic aorta gene expression in spontaneous hypertensive rats. Evid Based Complement Alternat Med. 2014;2014:565784. doi:10.1155/2014/565784
136. Xie PC, Liang QE, Tu WQ, et al. The effect of Taohong Siwu decoction combined with antihypertensive medicine in the treatment of hypertension: meta-analysis. Medicine. 2022;101(49):e32133. doi:10.1097/MD.0000000000032133
137. Weng JQ, Li JB, Yuan MF, et al. Effects of Buyang Huanwu decoction on intestinal barrier, intestinal flora, and trimethylamine oxide in rats with heart failure. Chin J Integr Med. 2023;29(2):155–161. doi:10.1007/s11655-022-2898-z
138. Li H, Xie HB, Qi R, et al. Effect of regulation of intestinal flora with the Cheqianzi Cuduotang capsules on blood pressure in elderly patients with hypertension. Clin J Chin Med. 2019;11(11):15–19.
139. Qi YZ, Jiang YH, Jiang LY, et al. Effects of Eucommia ulmoides-Tribulus terrestris on intestinal microbiota in aged spontaneously hypertensive rats. Chin J Hypertens. 2019;5:454–462. doi:10.16439/j.cnki.1673-7245.2019.05.017
140. Xu X, Tian W, Duan W, et al. Quanduzhong capsules for the treatment of grade 1 hypertension patients with low-to-moderate risk: a multicenter, randomized, double-blind, placebo-controlled clinical trial. Front Pharmacol. 2023;13:1014410. doi:10.3389/fphar.2022.1014410
141. Yu X, Zhang X, Jin H, et al. Zhengganxifeng decoction affects gut microbiota and reduces blood pressure via renin-angiotensin system. Biol Pharm Bull. 2019;42(9):1482–1490. doi:10.1248/bpb.b19-00057
142. Wang Y, Zhang P, Li H, et al. Zhijing powder manages blood pressure by regulating PI3K/AKT signal pathway in hypertensive rats. Heliyon. 2023;9(1):e12777. doi:10.1016/j.heliyon.2022.e12777
143. Zhang Y, Zhang D, Bai X, et al. The impact of Traditional Chinese Medicine on mouse gut microbiota abundances and interactions based on Granger causality and pathway analysis. Front Microbiol. 2022;13:980082. doi:10.3389/fmicb.2022.980082
© 2024 The Author(s). This work is published and licensed by Dove Medical Press Limited. The full terms of this license are available at https://www.dovepress.com/terms.php and incorporate the Creative Commons Attribution - Non Commercial (unported, v3.0) License.
By accessing the work you hereby accept the Terms. Non-commercial uses of the work are permitted without any further permission from Dove Medical Press Limited, provided the work is properly attributed. For permission for commercial use of this work, please see paragraphs 4.2 and 5 of our Terms.