Back to Journals » International Journal of Nanomedicine » Volume 19
Stem Cell-Derived Extracellular Vesicles: Promising Therapeutic Opportunities for Diabetic Wound Healing
Authors Zhang B, Bi Y , Wang K, Guo X, Liu Z , Li J, Wu M
Received 25 January 2024
Accepted for publication 10 May 2024
Published 17 May 2024 Volume 2024:19 Pages 4357—4375
DOI https://doi.org/10.2147/IJN.S461342
Checked for plagiarism Yes
Review by Single anonymous peer review
Peer reviewer comments 4
Editor who approved publication: Professor Lijie Grace Zhang
Boyu Zhang,1,* Yajun Bi,2,* Kang Wang,1,* Xingjun Guo,3 Zeming Liu,1 Jia Li,1 Min Wu1
1Department of Plastic and Cosmetic Surgery, Tongji Hospital, Tongji Medical College, Huazhong University of Science and Technology, Wuhan, 430030, People’s Republic of China; 2Department of Pediatrics, Dalian Municipal Women and Children’s Medical Center (Group), Dalian Medical University, Dalian, Liaoning Province, 116011, People’s Republic of China; 3Department of Biliary-Pancreatic Surgery, Affiliated Tongji Hospital, Tongji Medical College, Huazhong University of Science and Technology, Wuhan, Hubei, 430030, People’s Republic of China
*These authors contributed equally to this work
Correspondence: Jia Li; Min Wu, Department of Plastic and Cosmetic Surgery, Tongji Hospital, Tongji Medical College 1095 Jiefang Avenue, Wuhan, Hubei, People’s Republic of China, Tel +86 18846818658 ; +86 13554254796, Email [email protected]; [email protected]
Abstract: Wound healing is a sophisticated and orderly process of cellular interactions in which the body restores tissue architecture and functionality following injury. Healing of chronic diabetic wounds is difficult due to impaired blood circulation, a reduced immune response, and disrupted cellular repair mechanisms, which are often associated with diabetes. Stem cell-derived extracellular vesicles (SC-EVs) hold the regenerative potential, encapsulating a diverse cargo of proteins, RNAs, and cytokines, presenting a safe, bioactivity, and less ethical issues than other treatments. SC-EVs orchestrate multiple regenerative processes by modulating cellular communication, increasing angiogenesis, and promoting the recruitment and differentiation of progenitor cells, thereby potentiating the reparative milieu for diabetic wound healing. Therefore, this review investigated the effects and mechanisms of EVs from various stem cells in diabetic wound healing, as well as their limitations and challenges. Continued exploration of SC-EVs has the potential to revolutionize diabetic wound care.
Keywords: diabetes, wound healing, stem cells, EVs, ncRNAs, regeneration
Graphical Abstract:
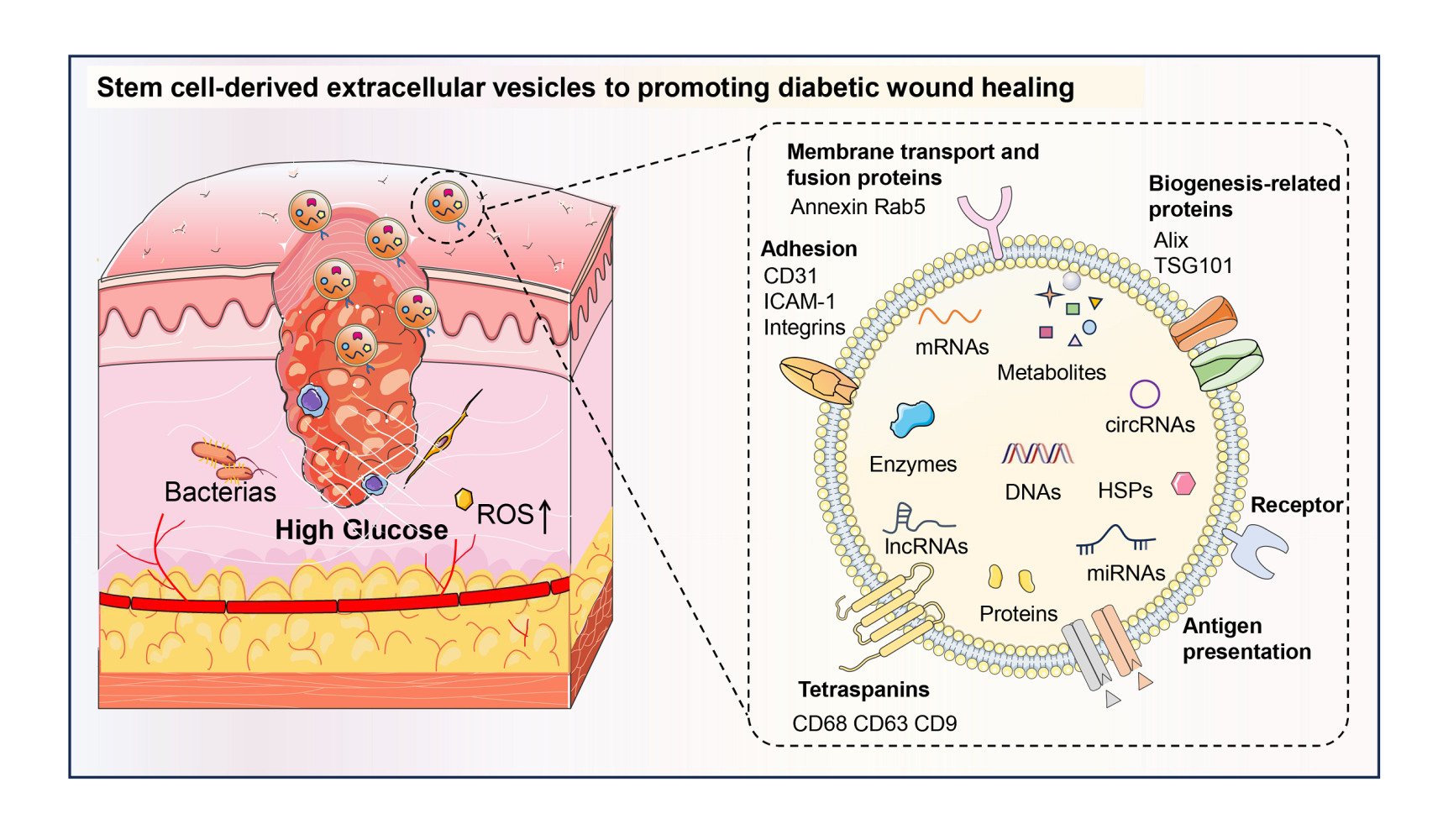
Introduction
The skin is the largest organ of the body and is extremely vulnerable to disruption and impairment by various factors.1 Repairing skin wound damage is extremely important for maintaining the integrity and functionality of the skin. However, cutaneous wound healing involves a series of successive, overlapping, and sophisticated processes involving crosstalk and coordinated interactions among multiple cell types and can be divided into four phases: hemostasis, inflammation, proliferation, and tissue remodeling.2
Diabetes mellitus (DM) is a persistent metabolic disease with progressively increasing morbidity and mortality rates that is characterized by chronically elevated blood glucose and results in disruption of the organic microenvironment.3–6
Importantly, the complications caused by diabetes cannot be ignored. Refractory cutaneous wounds, represented by diabetic foot ulcers (DFUs), are a typical complication characterized by an adverse inflammatory response, impaired angiogenesis, and disturbed extracellular matrix (ECM) remodeling7 (Figure 1). These complications can cause severe clinical outcomes, disability, deformity, and even mortality, thus creating a major public health burden. Therefore, exploring novel programmatic strategies for diabetic wound healing has practical clinical value.
Stem cells are undifferentiated cells that exhibit multiple functions, such as multidirectional differentiation, self-renewal potential, and paracrine bioactivity.8 Stem cells are abundant in natural sources, such as bone marrow, fat, placenta, and epidermis.9 Stem cell-based remedies have been extensively documented for neurological, circulatory, orthopedic, and other systemic diseases.10–12 However, stem cell therapies still face major controversies and challenges, including low survival rates, inevitable risks of tumors, and ethical issues associated with donor sources.13
Currently, exocrine derivatives have attracted increased attention as the primary substances that mediate the functional activity of stem cells. Extracellular vesicles (EVs), the donors of which can be almost any type of cell, are secreted into the extracellular space in the form of membrane vesicles. EVs can be absorbed and internalized by recipient cells.14 EVs serve as important mediators of intercellular communication and molecular crosstalk and are deeply involved in various physiopathological processes in individual organisms.15 Remarkably, stem cell-derived EVs map to parental cells, resulting in increased safety and local survival and decreased ethical and embolic risks during the therapeutic process16 (Figure 2). Stem cell-derived extracellular vesicles (SC-EVs) contain many non-coding RNAs (ncRNAs), DNA molecules, proteins, and metabolites that stimulate and recruit downstream molecules and effector cells to induce a series of cascading responses. Specifically, EVs can result in a multilayered restoration through various positive effects, including the modulation of immune polarization, suppression of oxidative stress-induced damage, anti-inflammatory effects, revascularization, endothelial regeneration, and promotion of collagen remodeling during wound healing.17 Furthermore, SC-EVs possess superior plasticity and expandability. Pretreating the original stem cells with pharmacological and physical measures or by the targeted editing of the effective components in EVs can substantially increase the therapeutic efficacy of EVs.18
SC-EVs, are emerging as potent facilitators of wound healing due to their nanoscale size, allowing for deep tissue penetration and direct interactions with target cells. SC-EVs are packed with a variety of bioactive molecules such as growth factors, signaling molecules, and nucleic acids inherited from their parent cells. These components are crucial for promoting key healing processes, including cell proliferation, angiogenesis, and tissue remodeling. The adaptability of stem cell-derived EVs is particularly noteworthy. SC-EVs can be functionally and compositionally tuned to respond to various stimuli, enhancing their therapeutic efficacy. This tunability allows for targeted delivery and modification, tailoring the SC-EVs to meet specific healing needs and improving their integration into cellular repair networks. As natural mediators of intercellular communication, SC-EVs facilitate bidirectional interactions with cells in the wound, strengthening cellular repair effects and accelerating the healing process. In summary, SC-EVs are significant candidates for optimizing wound healing by delivering essential bioactive molecules, enhancing cellular communication, and adapting to the dynamic wound environment. Therefore, this review focuses on the detailed mechanisms of diverse categories of SC-EVs in treating diabetic wound healing, intending to provide novel options for wound healing therapy.
The Mechanism of Stem Cell-EVs in Diabetic Wound Healing
ADSCs
Among adult stem cells, adipose-derived stem cells (ADSCs) possess considerable potential for utilization in cell therapy and tissue engineering. This potential is attributed to easy accessibility, active secretory activities, and immunomodulatory capacity.19 Recently, ADSC-derived EVs (ADSC-EVs) are paramount paracrine activators of ADSCs for retaining the function of the original cells with lower immunogenicity and deeper applications.20 In particular, ADSC-EVs are considered robust candidates for use in diabetic wound healing because of their potential functions of regulating cell proliferation and apoptosis and participating in angiogenesis, immunomodulation, and ECM remodeling.21 It is worth noting that gene editing technology offers the possibility of amplifying the characteristic substances and functional synergies of cells.22–25
EVs from ADSCs
ADSC-EVs are mapped derivatives of parental cells with the ability to alleviate the negative effects of oxidative stress and have anti-inflammatory characteristics.26 Xiao et al found that ADSC-derived exosomes (ADSC-exos) could upregulate SIRT3 expression and strengthen the activity of SOD2, which alleviated oxidative stress and inflammatory response and promoted vessel formation, thereby achieving remarkable healing efficacy in chronic diabetic wounds.27 Besides, the binding of HSP90 on the surface of ADSC-exos binding to the LRP1 receptor could stimulate the activation of the AKT signaling pathway, thereby reducing hypoxia and oxidative stress damage induced by high glucose(HG).28 ADSC-EVs probably provided a novel treatment for enhancing diabetic corneal epithelial wounds based on the efficient anti-inflammatory effects. Wang et al found that ADSC-EVs decreased the level of pro-inflammatory cytokines such as IL-6, TNF-α, and IL-1β, caused by dendritic cells (DCs) through activation of the NGF/TrkA pathway.29
ADSC-EVs can be absorbed by dermal fibroblasts (FBs) and promote their physiological activity, mediating collagen deposition and matrix remodeling.26 Zhao et al demonstrated that ADSC-exos strengthened the proliferative, migratory, and invasive capabilities of human dermal fibroblast WS1 cells in vitro. Furthermore, ADSC-exos accelerated wound healing in db/db mice by downregulating MMP-1 and MMP-3 expression, thus promoting collagen deposition.30 The secretion of MMP-9 is an important factor in the healing process. ADSC-EVs induced proliferation and MMP-9 secretion in HaCaT cells. In vivo animal model, ADSC-EVs accelerated epithelization and collagen enrichment to promote diabetic wound closure by inhibiting MMP-9 expression.31 Xiao et al demonstrated that in vitro, ADSC-exos increased the proliferative and migratory abilities of HDFs and promoted proliferation and tube formation in HUVECs. Additionally, these researchers combined ADSC-exos with human acellular amniotic membrane (hAAM) biomaterials, and hAAM exhibited favorable efficacy when combined with ADSC-exos. In addition, hAAM loaded with ADSC-exos effectively regulated inflammation, stimulated vascularization, and facilitated ECM deposition to promote diabetic wounds in vivo mouse model.32
TGF-β1, as a responsive molecule, participates in diabetic ADSC-EVs mediated cellular crosstalk and initiates wound regeneration in the early stage.33 Xu et al found that ADSC-exos could induce macrophages to activate the TGF-β/Smad3 signaling pathway via increased TGF-β1 secretion. Besides, TGF-β1 could activate the FBs in an autocrine manner to increase TGF-β1 production, thus promoting ECM deposition and re-epithelization leading to the healing of diabetic wounds.34
The unique features of EVs can be fully demonstrated by rescuing AGE-mimicked disorders of diabetic microenvironments in vitro. Wang et al discovered that ADSC-exos assisted in the recovery of diabetic wounds by suppressing the apoptosis and promoting re-epithelialization and angiogenesis of keratinocytes (KCs) conditioned with advanced glycation end-product (AGE) through inhibiting Fas/Fasl signaling pathway.35 Similarly, Liu et al ADSC-EVs substantiated that exhibited capabilities in promoting HIF-1α mediated angiogenesis proliferation, migration, and tube formation of AGE-treated HUVECs. Specifically, the activation of hypoxia-related PI3K/AKT/mTOR signaling pathway induced HIF-1α and vascular endothelial growth factor (VEGF) upregulation to incorporate these promotion effects.36
EV-Derived miRNAs from ADSCs
MicroRNAs (miRNAs) are a group of small ncRNAs operating in the post-transcriptional regulation of gene expression and efficiently regulate cell growth, differentiation, and apoptosis among various cellular activities.37,38 ADSC-EVs contain various miRNAs with several excellent properties, such as physicochemical stability and multidimensional packaging properties. And these miRNAs from ADSC-EVs are also modified and customized by the original cells.39 Based on these natural dominances, Lv et al found that ADSC-exos with miR-21-overexpression exerted a potent effect in boosting KC proliferation by activating Wnt/β-catenin signaling in vitro, and thus further alleviating diabetic wounds, accompanied by re-epithelialization, collagen synthesis, neovascularization, and vessel maturation.40 Moreover, Ge et al explored that miR-132-overexpressing ADSC-exos enhanced the healing of diabetic wounds by mitigating regional inflammation, facilitating collagen production, promoting the microvascular generation, and stimulating the polarization of M2 macrophages by suppressing the NF-κB pathway.41
EV-Derived lncRNAs from ADSCs
LncRNAs are a type of ncRNA that are located in the nucleus and cytoplasm of eukaryotic cells and are more than 200 bp in length.42 LncRNAs have regulatory functions in cell proliferation, invasion, metastasis, apoptosis, and immune response, which are accomplished through epigenetic modification, transcriptional regulation, and translational regulation.43 LncRNAs are inextricably linked to the regulation of cellular functions and critical biological pathways, demonstrating their potential in diabetic wound healing. The overexpression of linc00511 in ADSC-exos demonstrated competency in DFU healing through inducing angiogenesis, which was attributed to suppressing PAQR3-induced Twist1 ubiquitination degradation.44 Apart from this, lncRNA MALAT1 in ADSC-exos downregulated the expression of miR-378a and upregulated downstream FGF2, thus enhancing the proliferative and migratory ability of human skin fibroblasts (HSFs) and offering a potential therapeutic target for diabetic wound healing.45
EV-Derived circRNAs from ADSCs
Circular RNAs (circRNAs) belong to the family of ncRNAs that are linked in an end-to-end manner to form single-stranded circular molecules with covalently closed forms. CircRNAs are widespread in natural species and are commonly known for their diversity, evolutionary conservation, stability, and specificity.46 Wang et al showed that high expression of circ-Astn1 in ADSC-exos decreased apoptosis and promoted angiogenesis in endothelial precursor cells (EPCs) induced by HG and promoted diabetic ulcers via the miR-138-5p/SIRT1/FOXO1 signaling pathway. This study posed a novel circ-Astn1/miR-138-5p/SIRT1 axis as a therapeutic target.47
Further studies demonstrated that mmu_circ_0001052 and mmu_circ_0000250 served as inducers involved in the angiogenesis of endothelial cells (ECs) in HG environments. The mmu_circ_0001052-modified ADSC-exos regulated the miR-106a-5p and FGF4/p38MAPK axis, thereby promoting proliferation, migration, and angiogenesis of HUVECs treated with HG, which in turn improved wound healing in DFU mice.48 In addition, the high level of mmu_circ_0000250 in ADSC-exos promoted the uptake of miR-128-3p, which led to the upregulation of SIRT1 and subsequent activation of autophagy, strengthening the therapeutic efficacy of exosomal mmu_circ_0000250/miR-128-3p/SIRT1 in diabetic wound healing.49 HIF-1α possesses critical functions in the promotion of angiogenesis and VEGF secretion and is regarded as the pivotal switch in diabetic wound healing.50 CircRNAs synergistically contribute to the healing effect via up-regulating downstream HIF-1α content. Wang et al found that Circ-Gcap14 from hypoxia-preconditioned ADSC-exos showed the potential in expediting diabetic wound healing. This function can be achieved by circ-Gcap14 down-regulating miR-18a-5p and further promoting downstream HIF-1α expression.51 In contrast, circ-Snhg11-overexpressing ADSC-EVs inhibited HG-induced EC injury and triggered M2 macrophage polarization via the miR-144-3p/HIF-1α axis, and these effects ultimately caused the healing of diabetic wounds.52
EV-Derived Proteins from ADSCs
Nuclear factor erythroid 2-related factor 2 (Nrf2) exhibits a positive response to exogenous and endogenous oxidative stress, which represents a valid target for diabetic wound healing.53 Li et al showed that Nrf2-overexpressing ADSC-exos effectively prevented EPC senescence by inhibiting reactive oxygen species (ROS) and the inflammatory cytokines IL-1β, IL-6, and TNF-α. Consequently, Nrf2-overexpressing ADSC-exos were shown to contribute to accelerated vascularization in diabetic rat wounds.54 Nuclear factor I/C (NFIC) belongs to the factor I family, which is involved in the modulation of diabetes-related diseases, such as diabetic nephropathy.55 However, the effectiveness of this molecule in treating diabetic wounds has not been adequately validated. Huang et al reported that the overexpression of NFIC in ADSC-exos could revise HG-induced HUVEC injury by promoting miR-204-3p expression and further triggering the downstream HIPK2 suppression. Therefore, NFIC in ADSC-exos ameliorated DFU by modulating the miR-204-3p/HIPK2 axis.56 Previous studies have shown that ginsenoside (Rg-1) can upregulate the expression of IRF1 to promote wound closure in DFUs.57,58 Interestingly, Wu et al reported that IRF1-overexpressing ADSC-exos was able to strengthen the proliferation and migration of FBs as well as the angiogenesis ability of ECs via the regulation of the miR-16-5p/SP5 axis, thus facilitating the DFU wound healing.59 HIF-1α is a cardinal regulator-induced cellular adaptation to hypoxia and wound healing promoter.60 Consequently, HIF-1α-overexpressing ADSC-exos significantly improved the proliferation and migration of FBs and the formation of ECM in vitro. These modified exosomes also inhibited early inflammation by activating the PI3K/AKT pathway, thus achieving ideal diabetic wound healing.61
Pretreatment with ADSC-EVs
MSCs subjected to various preconditioning methods in vitro showed effective activation of diverse vital signaling pathways and rescue of their loss of function. Consequently, pretreatment approaches can improve the therapeutic efficacy of MSCs in tissue engineering and regenerative medicine.62 Further research illustrated that pretreated stem cells showed superior paracrine effects and hence strengthened the impact of ADSC-EVs on the healing of diabetic wounds. For instance, low-intensity ultrasound could enhance the generation of wound healing-related miRNAs in ADSC-exos, including let-7b-5p, miR-17-5p, miR-99b-3p, miR-106b-3p, miR-140-3p, miR-143-5p, miR-145-5p, miR-222-3p, miR-320a-3p, miR-423-5p, miR-493-3p, miR-589-5p, miR-94.Additionally, ultrasound-pretreated ADSC-exos could facilitate FB, KC, and EC proliferation and migration and increase diabetic wound healing in db/db mice in vivo through re-epithelialization, collagen production, and angiogenesis process.63 Whereas, Wang et al found that hypoxia-preconditioned ADSC-exos could enhance the secretion of vascular growth factors and ECM via PI3K/AKT pathway activation, thereby promoting fibroblast proliferation and migration ability. Additionally, these EVs could also upregulate inflammatory factors and chemokines to improve the velocity and efficiency of diabetic wound healing64 (Figure 3).
BMSCs
BMSCs are multipotent cells that can self-renew, differentiate into various cell types, and produce diverse biologically active molecules.65 BMSCs hold considerable potential for application in regenerative medicine. Extensive evidence suggests that BMSCs exhibit promising therapeutic strategies for wound healing and that EVs secreted by BMSCs offer excellent clinical prospects for the treatment of diabetic wounds due to their good biocompatibility and immutability.
EVs from BMSCs
ADSC-EVs can enhance the migration, proliferation, and regeneration of new vessels in vascular ECs. Similar to ADSC-EVs in wound healing, BMSC-EVs are effective accelerators of EPC tube formation in vitro and re-epithelization, collagen deposition, and neovascularization in diabetic rats in vivo, which could be utilized for treating diabetic wounds synergistically with small molecule Nrf2 activator tert-butylhydroquinone (tBHQ).66
EV-Derived miRNAs from BMSCs
BMSC-EVs strengthened HaCaT cell migration, FGF-7 secretion, and anti-inflammatory effects and promoted re-epithelialization, angiogenesis, and collagen deposition in mouse models in vivo, which effectively promoted diabetic wound healing.67 This therapeutic effect is linked to miR-155, which is one of the earliest identified miRNAs. And miR-155 is recognized as a regulator of the immune system and inflammation and is sensitive to the stimulation of inflammation-related signaling pathways.68
EV-Derived lncRNAs from BMSCs
LncRNAs are known to play important roles in mediating cell proliferation, migration, inflammation, apoptosis, and autophagy.69 Several studies have shown that lncRNAs from BMSC-EVs are involved in various stages of diabetic wound healing by regulating diabetic wound healing. LncRNA H19 from BMSC-exos promoted fibroblast proliferation and migration, rescued fibroblast apoptosis and inflammation by inhibition of miR-152-3p, which in turn upregulated the expression of PTEN, thus accordingly offering an effective therapeutic option for the healing of DFUs.70 And lncRNA KLF3-AS1 derived from BMSC-exos protected the biological function of HUVECs from HG damage and promoted neovascularization to expedite diabetic wound healing in full-thickness wounds of diabetic mice through downregulating miR-383 and VEGFA secretion.71 Further research has demonstrated that the overexpression of lncRNA HOTAIR in BMSC-EVs could increase VEGFA content in ECs, and reinforce the local angiogenesis effect to accelerate wound healing in diabetic mice in vivo.72
EV-Derived circRNAs from BMSCs
CircRNAs participate in cell growth, migration, invasion, and apoptosis. Circ-ITCH, a renowned circRNA that spans several exons of the itch E3 ubiquitin ligase (ITCH), plays a role in various diseases by sponging specific miRNAs.73 Chen et al studied that circ-ITCH from BMSC-exos could specifically bind to TAF15 protein and induce Nrf2 pathway activation. This process restrained ferroptosis and promoted HUVEC angiogenesis, thus providing an exotic perspective to accelerate DFU wound healing.74
Pretreated BMSC-EVs
Research has demonstrated that pretreatment can enhance the survival and biological function of MSCs in the transplantation microenvironment, presenting novel ideas and possibilities for the treatment of diabetic wounds.75 The pretreatment of BMSC-EVs through cell culture with melatonin, pioglitazone, and atorvastatin (ATV) has yielded notable outcomes in advancing the healing of diabetic wounds. Liu et al found that melatonin-pretreated BMSC-exos activated the PTEN/AKT signaling pathway to increase the M2/M1 polarization ratio in vitro and in animal models in vivo to inhibit inflammatory responses and facilitate diabetic wound healing.76 Yu et al reported that atorvastatin-pretreated BMSC-exos promoted the vascularization of ECs in an in vivo rat model by upregulating miR-221-3p expression via the AKT/eNOS pathway, providing a potent therapeutic method for facilitating the healing of diabetic wounds.77 Hu et al reported that pioglitazone-treated BMSC-exos restored the proliferation and angiogenesis of HG-injured HUVECs and increased their vascularization in vivo in a rat model by activating the PI3K/AKT/eNOS pathway, thereby facilitating diabetic wound healing.78 Besides, IFN-γ-pretreated BMSC-exos induced strong miR-126-3p enrichment, which promoted the biological function of HUVECs, and ameliorated diabetic wounds mainly via the SPRED1/Ras/Erk axis in an in vivo physiological model using mouse angiogenesis.79 These results provide a solid therapeutic basis for the use of BMSC-EVs to promote diabetic skin regeneration and repair.
PMSCs
Placentas are promising sources of MSCs due to their abundance and easy accessibility. PMSCs have proliferative, migratory, clonal, and immunomodulatory potential, so these PMSCs have broad applications in the clinical field.80 PMSCs own the capacity to secrete plenty of cytokines and chemokines being tightly associated with angiogenic signal transduction.81 These properties make PMSCs an advantageous weapon in the proangiogenic process. PMSCs include MSCs from different placental tissue sections, such as human amniotic epithelial cells (hAECs) and human umbilical cord mesenchymal stem cells (hUC-MSCs) among others.
hUC-MSCs
EVs from hUC-MSCs
EVs from hUC-MSCs enter the interior of effector cells by endocytosis. Thus, these exos can modulate cell functions and increase the remediation efficacy during all stages of the wound healing process. Teng et al reported that hUC-MSC-exos promoted the proliferation of HUVECs and NIH-3T3 cells in vitro and accelerated diabetic wound healing in diabetic rats in vivo by facilitating M2 macrophage polarization, angiogenesis, and collagen deposition.82 Furthermore, hUC-MSC-exos effectively suppressed HG-induced oxidative stress damage and increased angiogenesis and proliferation in HUVECs, accelerating diabetic skin wound healing both in vitro and in vivo in diabetic mouse models.83 Liu et al innovatively constructed cell aggregates (CAs) from hUC-MSCs based on specific culture and cell sheet engineering technology, which could prevent cell volume loss due to digestion, preserve cell-to-cell interactions, and possess more potent secretion power. CD31+EMCN+ vessels, a specific vascular subtypephenotypically were certified the lower density and impaired regeneration in mice with type 2 diabetes (T2D).84 These researchers further explored whether EVs from hUC-MSC-CAs stimulated CD31+EMCN+ blood vessel regeneration through activating Notch signaling and increasing the levels of proteins associated with angiogenesis, thus identifying a novel candidate for diabetic wound healing.
EV-Derived miRNAs from hUC-MSCs
HUC-MSCs transmit micromolecular miRNAs in the form of EVs and modulate downstream cascading signal transduction variations correlated with tissue restoration in variable mechanisms. Ti et al demonstrated that high expression of let-7b in LPS-pretreated hUC-MSC-exos effectively induced a phenotypic shift from M1 to anti-inflammatory M2 macrophages by regulating the TLR4/NF-κB/STAT3/AKT signaling pathway to alleviate diabetic wounds.85 Similarly, Wei et al discovered that a high content of miR-17-5p in hUC-MSC-exos promoted the proliferation, migration, and angiogenic activity of HUVECs. In addition, exosomal miR-17-5p could down-regulated PTEN via activating the AKT/HIF-1α/VEGF signaling pathway to promote angiogenesis, thus contributing to the healing of diabetic wounds.86
EV-Derived Proteins from hUC-MSCs
Endothelial nitric oxide synthase (eNOS) is regarded as a rate-limiting enzyme for the induction of NO synthesis.87 Several studies have shown that NO is a crucial switch in the angiogenic process. Mice deficient in the eNOS gene exhibit delayed wound closure and impaired new vascular capillary growth.88 Therefore, Zhao et al discovered that eNOS-loaded hUC-MSC-exos under blue light irradiation promoted tissue repair and reduced inflammatory cell infiltration and inflammation in diabetic wounds.89 These EVs could promote diabetic wound tissue regeneration through activation of the PI3K/AKT/mTOR and FAK/ERK1/2 pathways and induction of autophagy.
Pretreated hUC-MSCs
Nocardia rubra cell wall skeleton (Nr-CWS) can effectively activate macrophages and promote angiogenesis, thereby further accelerating skin wound healing.90 Qiang et al exploratively utilized the Nr-CWS-pretreated hUC-MSCs to extract EVs contributing to the proliferation, migration, and tubular structure formation of HG-injured HUVECs in vitro. Furthermore, these EVs promoted angiogenesis in animal models in vivo through the circIARS1/miR-4782-5p/VEGFA axis, which ultimately promoted diabetic wound healing.91
hAMSCs
The superior advantages of human amniotic membrane-derived mesenchymal stem cells (hAMSCs) are their multilineage differentiation, less tumourigenicity, and inhibition of inflammation.92 hAMSC-secreted EVs contain multiple lncRNAs. Fu et al reported that several lncRNAs associated with neovascularization were included in hAMSC-exos such as PANTR1, H19, OIP5-AS1, and NR2F1-AS1. These exosomal lncRNAs could promote diabetic wound healing through angiogenesis.93 In addition to this, it is also possible to reverse the biological abilities of FBs and HUVECs induced by HG damage in vitro as well as exert a pro-angiogenic effect to promote diabetic wound healing in vivo mouse models, which was mechanically through the activation of PI3K/AKT/mTOR pathway.94
EPCs
Endothelial progenitor cells (EPCs) are viewed as the precursor cells of vascular ECs and are promoters of damaged blood vessel reparation and generate radioactive factors such as VEGF, which play an essential role in the course of vascular genesis and development.95 Li et al studied that EPC-exos increased the proliferation, migration, and tube formation function of vascular ECs in vitro by enhancing the synthesis of pro-angiogenic molecules, thus representing a promising therapeutic approach for diabetic wound healing.96 Besides, Zhang et al found that EPC-exos significantly increased vasculogenic capacity in vitro and distinctly promoted diabetic cutaneous wound regeneration. These researchers further confirmed that Erk1/2 signaling activation was a critical mechanism in the process.97 In contrast, Xu et al found that EVs positively regulate cellular function through certainly enriched miRNAs by analyzing the miRNAs contained in EVs, such as miRNA-221-3p, one of the miRNAs with high expression in EPC-exos, which was closely correlated with promoting diabetic skin wound healing through angiogenesis enhancement.98 Li et al verified that upregulated miR-182-5p containing EPC-exos improved the ability of proliferation, and migration, and inhibited apoptosis in HaCaT cells, accelerating the process of wound healing in diabetic mice by inhibiting PPARG expression.99 Furthermore, preprocessing can enhance the beneficial impact of EVs. For instance, astragaloside IV pretreatment could efficiently boost the secretion of EVs. Particularly, the abundant miR-126-3p in EPC-exos enhanced the proliferation and migration function of HUVECs via inhibiting pyroptosis achieved by VEGF/PI3K/AKT axis, which performed tremendous substantial value in promoting diabetic wound regeneration.100
ESCs
Stem cells located in the epidermis and hair follicles are called embryonic stem cells (ESCs) and have beneficial functions in the maintenance of adult skin homeostasis and hair growth, as well as for epidermal reparative regeneration after injury.101 EVs are characterized as a forceful tool for diabetic epithelial cell repair and as representative of the paracrine function of ESCs. Xu et al explored that ESC-exos alleviated the excessive autophagy-mediated HUVEC apoptosis under HG and facilitated angiogenesis, which was the mechanism through activating the miR200b-3p/SYDE1/RAS/ERK autophagy pathway.102 High-throughput sequencing data of small RNAs included in ESC-exos indicated that the overall proportion of microRNAs was greater than that in fibroblast EVs. Wang et al found that ESCs-exos mainly controlled the PI3K/AKT and TGF-β signaling pathways to achieve the positive regulatory function in fibroblast proliferation and migration and M2 macrophage polarization in vitro. In diabetic mouse wounds, ESC-exos significantly accelerated diabetic wound healing by inhibiting the inflammatory response and enhancing proliferative and vascularization.103 Yang et al found that enriched miR-203a-3p in ESC-exos targeted SOCS3 and induced downstream JAK2/STAT3 signaling pathway activation to stimulate M2 macrophage polarization. The mechanism was also demonstrated in vivo mouse models to provide a better therapeutic effect in diabetic wound healing.104
iPSCs
The generation of induced pluripotent stem cells (iPSCs) is a unique and productive process that can be simply summarized as reprogramming cells under appropriate culture conditions with inducible factors followed by the reprogramming of somatic cells into pluripotent stem cells.105 iPSCs have formidable traits similar to those of ESCs, possessing the self-renewal and multidirectional differentiation ability into many types of cells, even the cardiomyocytes.106 The innovation in iPSC technology pioneers a completely revolutionary paradigm for regenerative medicine, disease model establishment, and drug research. However, the biological functions of EVs as an iPSC derivation have not been fully demonstrated. Kobayashi et al found that iPSC-exos facilitated the migration and pro-angiogenesis effect of FBs. In vivo mouse models, iPSC-exos enhanced vascularization and nerve regeneration, which accelerated diabetic wound closure.107 Notably, iPSC-derived MSCs (iMSCs), as one of the emerging types of MSC providers, against the limitations of amplification restriction and donor variability, have a greater preponderance in scalability and potential for tissue reparation.108 Both the iMSCs and iPSCs simultaneously as novel sources of MSCs performed better donor compatibility and extensibility. However, compared with iMSCs, undifferentiated iPSCs are less expensive in terms of reproducibility and practicability. Levy et al compared iPSC-EVs to donor-matched iMSC-EVs and found that iPSC-EVs demonstrated a proximate pro-angiogenic capacity and a greater anti-inflammatory capacity in vitro. In addition, the preferred healing of diabetic wounds confirmed the curative potential of iPSC-EVs, which was functionally achieved through immunoregulation in the induction of anti-inflammatory macrophage phenotypes induction.109 Bioinspired nanovesicles (NVs) prepared through continuous cellular extrusion mode can be deemed as exosome mimetics that have comparable cell membranes and sizes. These new types of NVs are enriched with more proteins and RNAs than EVs, as well as exhibiting greater productivity.110 Zhang et al utilized iPSC differentiation into ECs in anticipation of gaining further pro-angiogenic efficacy and innovatively constructed NVs. These researchers found that DA-loaded NVs derived from iPSC-ECs could target the delivery of DA to ECs based on abundant CXCR4 on the membrane surface, and enhance the tube formation of ECs. Moreover, these DA-loaded NVs promoted angiogenesis, epidermis regeneration, and collagen deposition via the HIF-1α/VEGFA pathway, thus accelerating diabetic wound healing.111
hFSCs
The hair follicles derived from the ectoderm, are the primary appendages of the skin. HFs are a workshop for stem cells and a germination center for hairs, with the function of remodeling the microenvironment of skin.112 Hair follicle mesenchymal stem cells (hFSCs) have the advantageous merits of being readily available, having high proliferative and differentiation capacity, and the lack of ethical arguments about stem cell origin, thereby making HF-MSCs the ideal candidates for cellular therapies and tissue engineering.113 HFSC-derived EVs (hFSC-EVs) have gradually become a promising option for skin rejuvenation and regeneration, and the reason is the inheritor of the dominant cellularity from provenance. Yang et al found that lncRNA H19 overexpression from HF-MSC-exos promoted HaCaT proliferation, migration, and inhibited apoptosis in vitro, and further enhanced diabetic skin wound healing in mouse models in vivo by reversing the stimulation of NLRP3 inflammasome-induced pyroptosis.114
USCs
Urinary-derived stem cells (USCs) obtained from a non-invasive collection method are excellent sources of transplantable cells for regenerative therapy and possess the advantages of high stemness properties and expandability, pluripotent differentiation potential, and immune-modulating potential. USCs can be used to repair epidermal and uroepithelial damage.115 Previous studies have validated DMBT1 as an important endothelial-derived ECM protein that is capable of binding angiogenic factors and contributing to adhesion, migration, proliferation, angiogenesis, and vascular repair.116 Chen et al discovered that DMBT1 in USC-derived exosomes could enhance the pro-angiogenic ability of ECs, and could also promote the formation of new blood vessels in vivo mouse models to expedite diabetic wound healing.117
MenSCs
Endometrial stem/progenitor cells from menstrual blood, which are identified as menstrual blood-derived mesenchymal stem cells (MenSCs), are effectively used in stem cell therapy based on their abundance, remarkable proliferation, and autologous transplantation capacity.118 Investigations of MenSC-derived EVs are still in the preliminary stages, Dalirfardouei et al discovered that MenSC-derived exosomes could lead to M1-M2 macrophage polarization to alleviate inflammatory reaction, and accelerated re-epithelialization by stimulating the NF-κB p65 subunit and NF-κB pathway, ultimately attenuating scar formation and reducing the collagen I: collagen III ratio, which might be a valuable tool in diabetic wound healing119 (Figure 4).
Challenges and Future Perspective
In summary, SC-EVs have regenerative potential; encapsulate diverse cargoes of proteins, RNAs, and cytokines; and exhibit safety, bioactivity, and few ethical issues. SC-EVs intricately coordinate organized regenerative processes by modulating intercellular communication, amplifying angiogenic responses, and facilitating the recruitment and differentiation of progenitor cells. The orchestrated mechanisms synergistically promote the healing milieu crucial for diabetic wound repair.
First, diabetic wound healing is a complex repair process involving multiple cells and factors, encompassing multiple stages. Theoretically, SC-EVs could exert bioactive effects on nearly all skin cells, and consequently advance wound healing. However, previous studies have primarily focused on the effects and mechanisms of SC-EVs on specific types or individual skin cells, without a detailed analysis of their function across all cells. This might lead to a lack of reasonable analysis of which skin cells SC-EVs have a dominant effect on. Then, wound healing encompasses four distinct yet overlapping phases. Existing studies typically focus on the end-stage effects and mechanisms of SC-EVs, while their roles and mechanisms during the early and middle stages of wound healing have not been fully elucidated. Moreover, analyses of the contents within SC-EVs have predominantly concentrated on ncRNAs and metabolites, which represent only a portion of the bioactive components carried by SC-EVs. Other components such as enzymes, lipids, and circRNAs, remain underexplored and warrant further investigation to optimize their effects on wound healing.120 It is worthwhile to emphasize the depth and breadth of SC-EVs in the future, explore the mechanistic effects of EVs on different systems and pathways from a holistic and multidimensional perspective, and construct a more ideal overall discursive approach.
Second, EVs are regarded as favorable candidates for cell-free regenerative medicine.121 Nevertheless, EVs still face several limitations and practical dilemmas in terms of storage, formulation, transportation, liberation, and overall mechanistic understanding.122–124 The establishment of standardized protocols, the implementation of scale-up strategies, and process optimization are essential prerequisites for the future advancement of applications. The separation methods currently used for EVs include ultracentrifugation, ultrafiltration, differential centrifugation, size chromatography exclusion, immunoaffinity, and polymer precipitation.125 Unfortunately, even though the extraction techniques are richly diverse, efficient, large-scale, and highly cost-effective procedures have yet to be established. Moreover, EVs are complicated mixtures of components, for which comprehensive and universally trustworthy characterization, as well as the complexity of transportation and storage, are challenges that cannot be ignored.126 The guidelines for quality control of EVs, as a new cutting-edge biological agent, and the related management of biosafety are not well established.127 The establishment of a novel, comprehensive concept for EV preparation is essential, with large-scale parent cell culture systems, suitable stem cell sources, stable contamination-free culture conditions, and the genetic stability of donor cells being potential key breakthrough points to increase the abundance of EVs. Future research will be dedicated to optimizing the preparation process for a more efficient, sustainable, and holistically integrated model for producing EV systems. This endeavor is expected to markedly increase both the quality and quantity of EV production, thereby advancing the development and application of this field.
Furthermore, worldwide applications for EVs in diagnostics and therapeutics have been expanding, while most related research has concentrated on preclinical studies with cellular and animal experiments, rarely reaching the level of clinical trials. However, the functions of EVs in the human microenvironment are largely unknown. Potential problems in the human body concerning the mechanisms of uptake, transformation, and excretion of EV systems have not yet been revealed. The security and underlying risks of EVs have yet to be evaluated.128 Given the broad understanding of the mechanisms of EVs in treating different diseases and the ongoing technological advancements, there is an expectation that EVs will exhibit a wider and more profound range of applications in human experiments.
Lastly, EV-based diabetic wound treatment presents many promising aspects. The three-dimensional cultivation pattern based on the combination of stem cells and structural scaffold materials, by mimicking the cell growth environment in vivo, is economical and practical in terms of time, space, manpower resources, and reagent consumables compared to the two-dimensional conventional cultivation pattern, with the prospect of a robust and reproducible standardized workflow for isolation.129 Moreover, biomaterials are used as loading and release systems for the exosomal system, both for their anti-inflammatory and antimicrobial properties and as physical scaffolds to imitate the internal matrix and to modulate the slow release of EVs by their self-degradation effect.123,130 Afterward, an efficient and feasible EV-biomaterial delivery system can be further developed to fully exploit the advantages of each and to provide a formidable experimental basis for future research on SC-EVs. Notably, SC-EVs offer a myriad of advantages and features in comparison to nanomedicine therapy and currently hold a prominent position in cancer treatment, drug delivery, diagnostics, and imaging. EVs are naturally released vesicles diverging from the typical artificial synthetic nature of nanomedicines and thereby exhibit superior biocompatibility, consequently ameliorating immune responses and toxicity.131 Additionally, SC-EVs can selectively target specific cells or tissues through surface membrane proteins or ligands, facilitating targeted drug delivery while sparing surrounding normal tissues from adverse effects.132 SC-EVs also harbor the capacity to mediate intercellular communication by transmitting signaling molecules, thereby regulating pathological processes.133 This novel delivery strategy has the potential for wider application, and its ability to promote the healing of diabetic wounds is unquestionable. The systematic integration of nanomaterials and exosomes provides a novel strategy for enhancing exosome activity.134–136 With a deeper understanding of the biological properties of EVs, there is an opportunity to further optimize their preparation methods and nanomedicine carrier functionality to improve their overall efficacy and biosafety. This optimization aimed to fully realize the potential of EVs in promoting wound healing in diabetic patients, paving the way for major advances in therapeutic interventions for diabetes-related wound care.
Conclusion
In summary, SC-EVs of diverse sources, ADSCs, BMSCs, PMSCs, EPCs, ESCs, iPSCs, hFSCs, USCs, and MenSCs, exert a vital role in regulating various cellular behaviors, including FBs, KCs, ECs, and immune cells (ICs), by preventing oxidative damage, accelerating vascular regeneration and re-epithelialization, and modulating immune responses and collagen remodeling (Table 1). SC-EVs are formidable vehicles for facilitating healing and tissue regeneration in diabetic wounds, thus expecting to be potential candidates for treating diabetic wounds.137,138
![]() |
Table 1 Application of Stem Cell-Derived Extracellular Vesicles in Diabetic Wound Healing |
Abbreviations
Adipose-derived mesenchymal stem cells (ADSCs); ADSC-derived EVs (ADSC-EVs); ADSC-derived exosomes (ADSC-exos); advanced glycation end-product (AGE); atorvastatin (ATV); bone marrow-derived mesenchymal stem cells (BMSCs); cell aggregates (CAs); circular RNAs (circRNAs); dendritic cells (DCs); diabetes mellitus (DM); diabetic foot ulcers (DFUs); embryonic stem cells (ESCs); endothelial cells (ECs); endothelial nitric oxide synthase (eNOS); endothelial precursor cells (EPCs); extracellular matrix (ECM); extracellular vesicles (EVs); fibroblasts (FBs); ginsenoside (Rg-1); hair follicle mesenchymal stem cells (hFSCs); hFSC-derived EVs (HF-MSC-EVs); high glucose (HG); human acellular amniotic membrane (hAAM); human amniotic epithelial cells (hAECs); human amniotic membrane-derived mesenchymal stem cells (hAMSCs); human skin fibroblasts (HSFs); human umbilical cord mesenchymal stem cells (hUC-MSCs); immune cells (ICs); induced pluripotent stem cells (iPSCs); iPSC-derived MSCs (iMSCs); itch E3 ubiquitin ligase (ITCH); keratinocytes (KCs); menstrual blood-derived mesenchymal stem cells (MenSCs); microRNAs (miRNAs); nanovesicles (NVs); Nocardia rubra cell wall skeleton (Nr-CWS); non-coding RNAs (ncRNAs); Nuclear factor erythroid 2-related factor 2 (Nrf2); nuclear factor I/C (NFIC); placenta-derived mesenchymal stem cells (PMSCs); reactive oxygen species (ROS); stem cell-derived extracellular vesicles (SC-EVs); tert-butylhydroquinone (tBHQ); type 2 diabetes (T2D); urinary-derived stem cells (USCs); vascular endothelial growth factor (VEGF).
Author Contributions
All authors made a major contribution to the work reported, whether in the conception, study design, execution, acquisition of data, analysis, and interpretation, or in all these areas; took part in drafting, revising or critically reviewing the article; gave final approval of the version to be published; agreed on the journal to which the article has been submitted; and agreed to be accountable for all aspects of the work.
Funding
This study was supported by financial support from the National Natural Science Foundation of China (grant number: 82202461), the Hubei Provincial Natural Science Foundation of China (grant numbers: 2021CFB043, 2022CFB226, and 2023AFB717), and the Research Foundation Project of Tongji Hospital (grant number: 2022A16).
Disclosure
The authors declare that they have no competing interests.
References
1. Grice EA, Segre JA. The skin microbiome. Nat Rev Microbiol. 2011;9(4):244–253. doi:10.1038/nrmicro2537
2. Mascharak S, Talbott HE, Januszyk M, et al. Multi-omic analysis reveals divergent molecular events in scarring and regenerative wound healing. Cell Stem Cell. 2022;29(2):315–327.e6. doi:10.1016/j.stem.2021.12.011
3. Zheng Y, Ma S, Huang Q, et al. Meta-Analysis of the Efficacy and Safety of Finerenone in Diabetic Kidney Disease. Kidney Blood Press Res. 2022;47(4):219–228. doi:10.1159/000521908
4. Cao J, Zhao C, Gong L, et al. MiR-181 Enhances Proliferative and Migratory Potentials of Retinal Endothelial Cells in Diabetic Retinopathy by Targeting KLF6. Curr Eye Res. 2022;47(6):882–888. doi:10.1080/02713683.2022.2039206
5. Li J, Wang X, Mao H, et al. Precision therapy for three Chinese families with maturity-onset diabetes of the young (MODY12). Front Endocrinol (Lausanne). 2022;13(13):858096. doi:10.3389/fendo.2022.858096
6. Zhu S, Li J, Zhao X. Comparative risk of new-onset hyperkalemia for antihypertensive drugs in patients with diabetic nephropathy: a Bayesian network meta-analysis. Int J Clin Pract. 2021;75(8). doi:10.1111/ijcp.13940
7. Chang M, Nguyen TT. Strategy for Treatment of Infected Diabetic Foot Ulcers. Acc Chem Res. 2021;54(5):1080–1093. doi:10.1021/acs.accounts.0c00864
8. Zakrzewski W, Dobrzyński M, Szymonowicz M, Rybak Z. Stem cells: past, present, and future. Stem Cell Res Ther. 2019;10(1):68. doi:10.1186/s13287-019-1165-5
9. Bacakova L, Zarubova J, Travnickova M, et al. Stem cells: their source, potency and use in regenerative therapies with focus on adipose-derived stem cells – a review. Biotechnol Adv. 2018;36(4):1111–1126. doi:10.1016/j.biotechadv.2018.03.011
10. Liu XY, Yang LP, Zhao L. Stem cell therapy for Alzheimer’s disease. World J Stem Cells. 2020;12(8):787–802. doi:10.4252/WJSC.V12.I8.787
11. Nguyen PK, Rhee JW, Wu JC. Adult stem cell therapy and heart failure, 2000 to 2016: a systematic review. JAMA Cardiol. 2016;1(7):831–841. doi:10.1001/jamacardio.2016.2225
12. Qi X, Zhang J, Yuan H, et al. Exosomes secreted by human-induced pluripotent stem cell-derived mesenchymal stem cells repair critical-sized bone defects through enhanced angiogenesis and osteogenesis in osteoporotic rats. Int J Biol Sci. 2016;12(7):836–849. doi:10.7150/ijbs.14809
13. Mazini L, Rochette L, Admou B, Amal S, Malka G. Hopes and Limits of Adipose-Derived Stem Cells (ADSCs) and Mesenchymal Stem Cells (MSCs) in Wound Healing. Int J Mol Sci. 2020;21(4):1306. doi:10.3390/ijms21041306
14. Jiang M, Jiang X, Li H, et al. The role of mesenchymal stem cell-derived EVs in diabetic wound healing. Front Immunol. 2023;14:1136098. doi:10.3389/fimmu.2023.1136098
15. Mulcahy LA, Pink RC, Carter DRF. Routes and mechanisms of extracellular vesicle uptake. J Extracell Vesicles. 2014;3. doi:10.3402/jev.v3.24641
16. Watanabe Y, Tsuchiya A, Terai S. The development of mesenchymal stem cell therapy in the present, and the perspective of cell-free therapy in the future. Clin Mol Hepatol. 2020;27(1):70–80. doi:10.3350/cmh.2020.0194
17. Zhou C, Zhang B, Yang Y, et al. Stem cell-derived exosomes: emerging therapeutic opportunities for wound healing. Stem Cell Res Ther. 2023;14(1):107. doi:10.1186/s13287-023-03345-0
18. Rong Y, Wang Z, Tang P, et al. Engineered extracellular vesicles for delivery of siRNA promoting targeted repair of traumatic spinal cord injury. Bioact Mater. 2023;23:328–342. doi:10.1016/j.bioactmat.2022.11.011
19. Li S, Sun J, Yang J, et al. Gelatin methacryloyl (GelMA) loaded with concentrated hypoxic pretreated adipose-derived mesenchymal stem cells(ADSCs) conditioned medium promotes wound healing and vascular regeneration in aged skin. Biomater Res. 2023;27(1):11. doi:10.1186/s40824-023-00352-3
20. Kou M, Huang L, Yang J, et al. Mesenchymal stem cell-derived extracellular vesicles for immunomodulation and regeneration: a next generation therapeutic tool? Cell Death Dis. 2022;13(7):580. doi:10.1038/s41419-022-05034-x
21. Deng H, Chen Y. The role of adipose-derived stem cells-derived extracellular vesicles in the treatment of diabetic foot ulcer: trends and prospects. Front Endocrinol (Lausanne). 2022;13:902130. doi:10.3389/fendo.2022.902130
22. Xu J, Chen Q, Tian K, et al. m6A methyltransferase METTL3 maintains colon cancer tumorigenicity by suppressing SOCS2 to promote cell proliferation. Oncol Rep. 2020;44(3):973–986. doi:10.3892/or.2020.7665
23. Ding L, Lu S, Zhou Y, et al. The 3′ Untranslated Region Protects the Heart from Angiotensin II-Induced Cardiac Dysfunction via AGGF1 Expression. Mol Ther. 2020;28(4):1119–1132. doi:10.1016/j.ymthe.2020.02.002
24. Xiong T, Xia L, Song Q. Circular RNA SPI1 expression before and after induction therapy and its correlation with clinical features, treatment response, and survival of acute myeloid leukemia patients. J Clin Lab Anal. 2023;37(3):e24835. doi:10.1002/jcla.24835
25. Duan F, Zeng W, Zhang Y, Li D, Wu K. Lipopolysaccharide enhances HSV-1 replication and inflammatory factor release in the ARPE-19 cells. Heliyon. 2022;8(11). doi:10.1016/j.heliyon.2022.e11787
26. Kong X, Patel NA, Chalfant CE, Cooper DR. Ceramide synthesis regulates biogenesis and packaging of exosomal MALAT1 from adipose derived stem cells, increases dermal fibroblast migration and mitochondrial function. Cell Commun Signal. 2023;21(1):221. doi:10.1186/s12964-022-00900-9
27. Zhang Y, Bai X, Shen K, et al. Exosomes Derived from Adipose Mesenchymal Stem Cells Promote Diabetic Chronic Wound Healing through SIRT3/SOD2. Cells. 2022;11(16):2568. doi:10.3390/cells11162568
28. Ren S, Chen J, Guo J, et al. Exosomes from Adipose Stem Cells Promote Diabetic Wound Healing through the eHSP90/LRP1/AKT Axis. Cells. 2022;11(20):3229. doi:10.3390/cells11203229
29. Wang G, Zeng L, Gong C, Gong X, Zhu T, Zhu Y. Extracellular vesicles derived from mouse adipose-derived mesenchymal stem cells promote diabetic corneal epithelial wound healing through NGF/TrkA pathway activation involving dendritic cells. Exp Eye Res. 2023;231:109484. doi:10.1016/j.exer.2023.109484
30. Zhao B, Zhang X, Zhang Y, et al. Human Exosomes Accelerate Cutaneous Wound Healing by Promoting Collagen Synthesis in a Diabetic Mouse Model. Stem Cells Dev. 2021;30(18):922–933. doi:10.1089/scd.2021.0100
31. Wang Wen J, Zhu Zheng Y, Hu X, et al. Extracellular Vesicles Derived from Adipose-Derived Stem Cells Accelerate Diabetic Wound Healing by Suppressing the Expression of Matrix Metalloproteinase-9. Curr Pharm Biotechnol. 2022;23(6):894–901. doi:10.2174/1389201022666210719154009
32. Xiao S, Xiao C, Miao Y, et al. Human acellular amniotic membrane incorporating exosomes from adipose-derived mesenchymal stem cells promotes diabetic wound healing. Stem Cell Res Ther. 2021;12(1):255. doi:10.1186/s13287-021-02333-6
33. Weng L, Ye J, Yang F, et al. TGF-β1/SMAD3 Regulates Programmed Cell Death 5 That Suppresses Cardiac Fibrosis Post-Myocardial Infarction by Inhibiting HDAC3. Circ Res. 2023;133(3):237–251. doi:10.1161/CIRCRESAHA.123.322596
34. Hsu HH, Wang AYL, Loh CYY, Pai AA, Kao HK. Therapeutic Potential of Exosomes Derived from Diabetic Adipose Stem Cells in Cutaneous Wound Healing of db/db Mice. Pharmaceutics. 2022;14(6):1206. doi:10.3390/pharmaceutics14061206
35. Wang JW, Zhu YZ, Ouyang JY, et al. Adipose-Derived Stem Cell Extracellular Vesicles Improve Wound Closure and Angiogenesis in Diabetic Mice. Plast Reconstr Surg. 2023;151(2):331–342. doi:10.1097/PRS.0000000000009840
36. Liu W, Yuan Y, Liu D. Extracellular Vesicles from Adipose-Derived Stem Cells Promote Diabetic Wound Healing via the PI3K-AKT-mTOR-HIF-1α Signaling Pathway. Tissue Eng Regen Med. 2021;18(6):1035–1044. doi:10.1007/s13770-021-00383-8
37. Li J, He D, Bi Y, Liu S. The Emerging Roles of Exosomal miRNAs in Breast Cancer Progression and Potential Clinical Applications. Breast Cancer Targets Ther. 2023;15:825–840. doi:10.2147/BCTT.S432750
38. Saliminejad K, Khorram Khorshid HR, Soleymani Fard S, Ghaffari SH. An overview of microRNAs: biology, functions, therapeutics, and analysis methods. J Cell Physiol. 2019;234(5):5451–5465. doi:10.1002/jcp.27486
39. Desdín-Micó G, Mittelbrunn M. Role of exosomes in the protection of cellular homeostasis. Cell Adhes Migr. 2017;11(2):127–134. doi:10.1080/19336918.2016.1251000
40. Lv Q, Deng J, Chen Y, Wang Y, Liu B, Liu J. Engineered Human Adipose Stem-Cell-Derived Exosomes Loaded with miR-21-5p to Promote Diabetic Cutaneous Wound Healing. Mol Pharm. 2020;17(5):1723–1733. doi:10.1021/acs.molpharmaceut.0c00177
41. Ge L, Wang K, Lin H, et al. Engineered exosomes derived from miR-132-overexpresssing adipose stem cells promoted diabetic wound healing and skin reconstruction. Front Bioeng Biotechnol. 2023;11:1129538. doi:10.3389/fbioe.2023.1129538
42. Zhang X, Wang W, Zhu W, et al. Mechanisms and functions of long non-coding RNAs at multiple regulatory levels. Int J Mol Sci. 2019;20(22):5573. doi:10.3390/ijms20225573
43. Yang Q, Fang D, Chen J, et al. LncRNAs associated with oxidative stress in diabetic wound healing: regulatory mechanisms and application prospects. Theranostics. 2023;13(11):3655–3674. doi:10.7150/thno.85823
44. Qiu J, Shu C, Li X, Ye C, Zhang WC. Exosomes from linc00511-overexpressing ADSCs accelerates angiogenesis in diabetic foot ulcers healing by suppressing PAQR3-induced Twist1 degradation. Diabet Res Clin Pract. 2021;180:109032. doi:10.1016/j.diabres.2021.109032
45. Pi L, Yang L, Fang BR. LncRNA MALAT1 from human adipose-derived stem cell exosomes accelerates wound healing via miR-378a/FGF2 axis. Regener Med. 2022;17(9):627–641. doi:10.2217/rme-2021-0170
46. He X, Xu T, Hu W, et al. Circular RNAs: their Role in the Pathogenesis and Orchestration of Breast Cancer. Front Cell Dev Biol. 2021;9:647736. doi:10.3389/fcell.2021.647736
47. Wang Z, Feng C, Liu H, et al. Exosomes from circ-Astn1-modified adipose-derived mesenchymal stem cells enhance wound healing through miR-138-5p/SIRT1/FOXO1 axis regulation. World J Stem Cells. 2023;15(5):476–489. doi:10.4252/wjsc.v15.i5.476
48. Liang ZH, Pan NF, Lin SS, et al. Exosomes from mmu_circ_0001052-modified adipose-derived stem cells promote angiogenesis of DFU via miR-106a-5p and FGF4/p38MAPK pathway. Stem Cell Res Ther. 2022;13(1):336. doi:10.1186/s13287-022-03015-7
49. Shi R, Jin Y, Hu W, et al. Exosomes derived from mmu_circ_0000250-modified adipose-derived mesenchymal stem cells promote wound healing in diabetic mice by inducing miR-128-3p/SIRT1-mediated autophagy. Am J Physiol Physiol. 2020;318(5):C848–C856. doi:10.1152/ajpcell.00041.2020
50. Zhang D, Lv FL, Wang GH. Effects of HIF-1α on diabetic retinopathy angiogenesis and VEGF expression. Eur Rev Med Pharmacol Sci. 2018;22(16):5071–5076. doi:10.26355/eurrev_201808_15699
51. Wang Z, Feng C, Liu H, et al. Hypoxic Pretreatment of Adipose-Derived Stem Cells Accelerates Diabetic Wound Healing via circ-Gcap14 and HIF-1α/VEGF Mediated Angiopoiesis. Int J Stem Cells. 2021;14(4):447–454. doi:10.15283/ijsc21050
52. Shi R, Jin Y, Zhao S, Yuan H, Shi J, Zhao H. Hypoxic ADSC-derived exosomes enhance wound healing in diabetic mice via delivery of circ-Snhg11 and induction of M2-like macrophage polarization. Biomed Pharmacother. 2022;153:113463. doi:10.1016/j.biopha.2022.113463
53. Long M, De La Vega MR, Wen Q, et al. An essential role of NRF2 in diabetic wound healing. Diabetes. 2016;65(3):780–793. doi:10.2337/db15-0564
54. Li X, Xie X, Lian W, et al. Exosomes from adipose-derived stem cells overexpressing Nrf2 accelerate cutaneous wound healing by promoting vascularization in a diabetic foot ulcer rat model. Exp Mol Med. 2018;50(4):1–14. doi:10.1038/s12276-018-0058-5
55. Zhang L, Zhang L, Li S, et al. Overexpression of MM9_CIRC_013935 alleviates renal inflammation and fibrosis in diabetic nephropathy via the MiR-153-3P/NFIC axis. Can J Physiol Pharmacol. 2021;99(11):1199–1206. doi:10.1139/cjpp-2021-0187
56. Huang H, Zhu W, Huang Z, Zhao D, Cao L, Gao X. Adipose-derived stem cell exosome NFIC improves diabetic foot ulcers by regulating miR-204-3p/HIPK2. J Orthop Surg Res. 2023;18(1):687. doi:10.1186/s13018-023-04165-x
57. Cai HA, Huang L, Zheng LJ, et al. Ginsenoside (Rg-1) promoted the wound closure of diabetic foot ulcer through iNOS elevation via miR-23a/IRF-1 axis. Life Sci. 2019;233:116525. doi:10.1016/j.lfs.2019.05.081
58. Zhou H, Jing S, Liu Y, et al. Identifying the key genes of Epstein–Barr virus-regulated tumour immune microenvironment of gastric carcinomas. Cell Prolif. 2023;56(3):e13373. doi:10.1111/cpr.13373
59. Wu M, Tu J, Huang J, Wen H, Zeng Y, Lu Y. Exosomal IRF1-loaded rat adipose-derived stem cell sheet contributes to wound healing in the diabetic foot ulcers. Mol Med. 2023;29(1):60. doi:10.1186/s10020-023-00617-6
60. Thangarajah H, Yao D, Chang EI, et al. The molecular basis for impaired hypoxia-induced VEGF expression in diabetic tissues. Proc Natl Acad Sci U S A. 2009;106(32):13505–13510. doi:10.1073/pnas.0906670106
61. Wang J, Wu H, Zhao Y, et al. Extracellular vesicles from hif-1α-overexpressing adipose-derived stem cells restore diabetic wounds through accelerated fibroblast proliferation and migration. Int J Nanomed. 2021;16:7943–7957. doi:10.2147/IJN.S335438
62. Hu C, Li L. Preconditioning influences mesenchymal stem cell properties in vitro and in vivo. J Cell Mol Med. 2018;22(3):1428–1442. doi:10.1111/jcmm.13492
63. Zheng Y, Xu P, Pan C, et al. Production and Biological Effects of Extracellular Vesicles from Adipose-Derived Stem Cells Were Markedly Increased by Low-Intensity Ultrasound Stimulation for Promoting Diabetic Wound Healing. Stem Cell Rev Rep. 2023;19(3):784–806. doi:10.1007/s12015-022-10487-w
64. Wang J, Wu H, Peng Y, et al. Hypoxia adipose stem cell-derived exosomes promote high-quality healing of diabetic wound involves activation of PI3K/Akt pathways. J Nanobiotechnology. 2021;19(1):202. doi:10.1186/s12951-021-00942-0
65. He X, Wang D, Yi Y, et al. δ-Tocotrienol preconditioning improves the capability of bone marrow-derived mesenchymal stem cells in promoting wound healing by inhibiting BACH1-related ferroptosis. Cell Death Discov. 2023;9(1):349. doi:10.1038/s41420-023-01653-1
66. Wang L, Cai Y, Zhang Q, Zhang Y. Pharmaceutical Activation of Nrf2 Accelerates Diabetic Wound Healing by Exosomes from Bone Marrow Mesenchymal Stem Cells. Int J Stem Cells. 2022;15(2):164–172. doi:10.15283/ijsc21067
67. Gondaliya P, Sayyed AA, Bhat P, et al. Mesenchymal Stem Cell-Derived Exosomes Loaded with miR-155 Inhibitor Ameliorate Diabetic Wound Healing. Mol Pharm. 2022;19(5):1294–1308. doi:10.1021/acs.molpharmaceut.1c00669
68. Xu WD, Feng SY, Huang AF. Role of miR-155 in inflammatory autoimmune diseases: a comprehensive review. Inflamm Res. 2022;71(12):1501–1517. doi:10.1007/s00011-022-01643-6
69. Li J, Tian H, Yang J, Gong Z. Long Noncoding RNAs Regulate Cell Growth, Proliferation, and Apoptosis. DNA Cell Biol. 2016;35(9):459–470. doi:10.1089/dna.2015.3187
70. Li B, Luan S, Chen J, et al. The MSC-Derived Exosomal lncRNA H19 Promotes Wound Healing in Diabetic Foot Ulcers by Upregulating PTEN via MicroRNA-152-3p. Mol Ther Nucleic Acids. 2020;19:814–826. doi:10.1016/j.omtn.2019.11.034
71. Han ZF, Cao JH, Liu ZY, Yang Z, Qi RX, Xu HL. Exosomal lncRNA KLF3-AS1 derived from bone marrow mesenchymal stem cells stimulates angiogenesis to promote diabetic cutaneous wound healing. Diabet Res Clin Pract. 2022;183:109126. doi:10.1016/j.diabres.2021.109126
72. Born LJ, Chang Hua K, Shoureshi P, et al. HOTAIR‐Loaded Mesenchymal Stem/Stromal Cell Extracellular Vesicles Enhance Angiogenesis and Wound Healing. Adv Healthc Mater. 2022;11(5):e2002070. doi:10.1002/adhm.202002070
73. Wang M, Chen B, Ru Z, Cong L. CircRNA circ-ITCH suppresses papillary thyroid cancer progression through miR-22-3p/CBL/β-catenin pathway. Biochem Biophys Res Commun. 2018;504(1):283–288. doi:10.1016/j.bbrc.2018.08.175
74. Chen J, Li X, Liu H, et al. Bone marrow stromal cell-derived exosomal circular RNA improves diabetic foot ulcer wound healing by activating the nuclear factor erythroid 2-related factor 2 pathway and inhibiting ferroptosis. Diabet Med. 2023;40(7):e15031. doi:10.1111/dme.15031
75. Li S, Yang K, Cao W, et al. Tanshinone IIA enhances the therapeutic efficacy of mesenchymal stem cells derived exosomes in myocardial ischemia/reperfusion injury via up-regulating miR-223-5p. J Control Release. 2023;358:13–26. doi:10.1016/j.jconrel.2023.04.014
76. Liu W, Yu M, Xie D, et al. Melatonin-stimulated MSC-derived exosomes improve diabetic wound healing through regulating macrophage M1 and M2 polarization by targeting the PTEN/AKT pathway. Stem Cell Res Ther. 2020;11(1):259. doi:10.1186/s13287-020-01756-x
77. Yu M, Liu W, Li J, et al. Exosomes derived from atorvastatin-pretreated MSC accelerate diabetic wound repair by enhancing angiogenesis via AKT/eNOS pathway. Stem Cell Res Ther. 2020;11(1):350. doi:10.1186/s13287-020-01824-2
78. Hu Y, Tao R, Chen L, et al. Exosomes derived from pioglitazone-pretreated MSCs accelerate diabetic wound healing through enhancing angiogenesis. J Nanobiotechnology. 2021;19(1):150. doi:10.1186/s12951-021-00894-5
79. Lu W, Du X, Zou S, et al. IFN-γ enhances the therapeutic efficacy of MSCs-derived exosome via miR-126-3p in diabetic wound healing by targeting SPRED1. J Diabetes. 2023. doi:10.1111/1753-0407.13465
80. Abumaree MH, Abomaray FM, Alshabibi MA, AlAskar AS, Kalionis B. Immunomodulatory properties of human placental mesenchymal stem/stromal cells. Placenta. 2017;59:87–95. doi:10.1016/j.placenta.2017.04.003
81. Li Q, Hu W, Huang Q, et al. MiR146a-loaded engineered exosomes released from silk fibroin patch promote diabetic wound healing by targeting IRAK1. Signal Transduct Target Ther. 2023;8(1):62. doi:10.1038/s41392-022-01263-w
82. Teng L, Maqsood M, Zhu M, et al. Exosomes Derived from Human Umbilical Cord Mesenchymal Stem Cells Accelerate Diabetic Wound Healing via Promoting M2 Macrophage Polarization, Angiogenesis, and Collagen Deposition. Int J Mol Sci. 2022;23(18):10421. doi:10.3390/ijms231810421
83. Yan C, Xv Y, Lin Z, et al. Human Umbilical Cord Mesenchymal Stem Cell-Derived Exosomes Accelerate Diabetic Wound Healing via Ameliorating Oxidative Stress and Promoting Angiogenesis. Front Bioeng Biotechnol. 2022;10:829868. doi:10.3389/fbioe.2022.829868
84. Liu L, Zheng CX, Zhao N, et al. Mesenchymal Stem Cell Aggregation-Released Extracellular Vesicles Induce CD31+EMCN+ Vessels in Skin Regeneration and Improve Diabetic Wound Healing. Adv Healthc Mater. 2023;12(20):e2300019. doi:10.1002/adhm.202300019
85. Ti D, Hao H, Tong C, et al. LPS-preconditioned mesenchymal stromal cells modify macrophage polarization for resolution of chronic inflammation via exosome-shuttled let-7b. J Transl Med. 2015;13:308. doi:10.1186/s12967-015-0642-6
86. Wei Q, Wang Y, Ma K, et al. Extracellular Vesicles from Human Umbilical Cord Mesenchymal Stem Cells Facilitate Diabetic Wound Healing Through MiR-17-5p-mediated Enhancement of Angiogenesis. Stem Cell Rev Rep. 2022;18(3):1025–1040. doi:10.1007/s12015-021-10176-0
87. Yamasaki K, Edington HDJ, McClosky C, et al. Reversal of impaired wound repair in iNOS-deficient mice by topical adenoviral-mediated iNOS gene transfer. J Clin Invest. 1998;101(5):967–971. doi:10.1172/JCI2067
88. Schwentker A, Billiar TR. Nitric oxide and wound repair. Surg Clin North Am. 2003;83(3):521–530. doi:10.1016/S0039-6109(02)00207-4
89. Zhao X, Fu L, Zou H, et al. Optogenetic engineered umbilical cord MSC-derived exosomes for remodeling of the immune microenvironment in diabetic wounds and the promotion of tissue repair. J Nanobiotechnology. 2023;21(1):176. doi:10.1186/s12951-023-01886-3
90. Hu K, Xu Y, Li X, Du P, Lu Y, Lyu G. The Nocardia Rubra Cell Wall Skeleton Regulates Macrophages and Promotes Wound Healing. Curr Issues Mol Biol. 2022;44(12):5995–6005. doi:10.3390/cimb44120408
91. Li Q, Guo L, Wang J, Tao S. Exosomes derived from Nr-CWS pretreated MSCs facilitate diabetic wound healing by promoting angiogenesis via the circIARS1/miR-4782-5p/VEGFA axis. Chin J Nat Med. 2023;21(3):172–184. doi:10.1016/S1875-5364(23)60419-4
92. Li JY, Ren KK, Zhang WJ, et al. Human amniotic mesenchymal stem cells and their paracrine factors promote wound healing by inhibiting heat stress-induced skin cell apoptosis and enhancing their proliferation through activating PI3K/AKT signaling pathway. Stem Cell Res Ther. 2019;10(1):247. doi:10.1186/s13287-019-1366-y
93. Fu S, Zhang H, Li X, et al. Exosomes Derived from Human Amniotic Mesenchymal Stem Cells Facilitate Diabetic Wound Healing by Angiogenesis and Enrich Multiple lncRNAs. Tissue Eng Regen Med. 2023;20(2):295–308. doi:10.1007/s13770-022-00513-w
94. Wei P, Zhong C, Yang X, et al. Exosomes derived from human amniotic epithelial cells accelerate diabetic wound healing via PI3K-AKT-mTOR-mediated promotion in angiogenesis and fibroblast function. Burn Trauma. 2020;8(tkaa020). doi:10.1093/BURNST/TKAA020
95. Balaji S, King A, Crombleholme TM, Keswani SG. The Role of Endothelial Progenitor Cells in Postnatal Vasculogenesis: implications for Therapeutic Neovascularization and Wound Healing. Adv Wound Care. 2013;2(6):283–295. doi:10.1089/wound.2012.0398
96. Li X, Jiang C, Zhao J. Human endothelial progenitor cells-derived exosomes accelerate cutaneous wound healing in diabetic rats by promoting endothelial function. J Diabetes Complications. 2016;30(6):986–992. doi:10.1016/j.jdiacomp.2016.05.009
97. Zhang J, Chen C, Hu B, et al. Exosomes derived from human endothelial progenitor cells accelerate cutaneous wound healing by promoting angiogenesis through Erk1/2 signaling. Int J Biol Sci. 2016;12(12):1472–1487. doi:10.7150/ijbs.15514
98. Xu J, Bai S, Cao Y, et al. MiRNA-221-3p in endothelial progenitor cell-derived exosomes accelerates skin wound healing in diabetic mice. Diabetes Metab Syndr Obes. 2020;13:1259–1270. doi:10.2147/DMSO.S243549
99. Li P, Hong G, Zhan W, et al. Endothelial progenitor cell derived exosomes mediated miR-182-5p delivery accelerate diabetic wound healing via down-regulating PPARG. Int J Med Sci. 2023;20(4):468–481. doi:10.7150/ijms.78790
100. Xiong W, Zhang X, Zhou J, et al. Astragaloside IV promotes exosome secretion of endothelial progenitor cells to regulate PI3KR2/SPRED1 signaling and inhibit pyroptosis of diabetic endothelial cells. Cytotherapy. 2023;S1465-3249(23):01045–01049. doi:10.1016/j.jcyt.2023.08.013
101. Blanpain C, Fuchs E. Epidermal stem cells of the skin. Annu Rev Cell Dev Biol. 2006;22:339–373. doi:10.1146/annurev.cellbio.22.010305.104357
102. Xu H, Yang H, Wang Z, et al. Epidermal stem cell derived exosomes alleviate excessive autophagy induced endothelial cell apoptosis by delivering miR200b-3p to diabetic wounds. J Invest Dermatol. 2023. doi:10.1016/j.jid.2023.08.030
103. Wang P, Theocharidis G, Vlachos IS, et al. Exosomes Derived from Epidermal Stem Cells Improve Diabetic Wound Healing. J Invest Dermatol. 2022;142(9):2508–2517.e13. doi:10.1016/j.jid.2022.01.030
104. Yang H, Xu H, Wang Z, et al. Analysis of miR-203a-3p/SOCS3-mediated induction of M2 macrophage polarization to promote diabetic wound healing based on epidermal stem cell-derived exosomes. Diabet Res Clin Pract. 2023;197:110573. doi:10.1016/j.diabres.2023.110573
105. Li G, Cheng G, Wu J, Ma S, Sun C. New iPSC for old long QT syndrome modeling: putting the evidence into perspective. Exp Biol Med. 2014;239(2):131–140. doi:10.1177/1535370213514000
106. Yoshida Y, Yamanaka S. Induced Pluripotent Stem Cells 10 Years Later: for Cardiac Applications. Circ Res. 2017;120(12):1958–1968.
107. Kobayashi H, Ebisawa K, Kambe M, et al. Effects of exosomes derived from the induced pluripotent stem cells on skin wound healing. Nagoya J Med Sci. 2018;80(2):141–153. doi:10.18999/nagjms.80.2.141
108. Yang H, Feng R, Fu Q, et al. Human induced pluripotent stem cell-derived mesenchymal stem cells promote healing via TNF-α-stimulated gene-6 in inflammatory bowel disease models. Cell Death Dis. 2019;10(10):718. doi:10.1038/s41419-019-1957-7
109. Levy D, Abadchi SN, Shababi N, et al. Induced Pluripotent Stem Cell-Derived Extracellular Vesicles Promote Wound Repair in a Diabetic Mouse Model via an Anti-Inflammatory Immunomodulatory Mechanism. Adv Healthc Mater. 2023;12(26):e2300879. doi:10.1002/adhm.202300879
110. Li YJ, Wu JY, Liu J, et al. Artificial exosomes for translational nanomedicine. J Nanobiotechnology. 2021;19(1):242. doi:10.1186/s12951-021-00986-2
111. Zhang W, Wang L, Guo H, Chen L, Huang X. Dapagliflozin-Loaded Exosome Mimetics Facilitate Diabetic Wound Healing by HIF-1α-Mediated Enhancement of Angiogenesis. Adv Healthc Mater. 2023;12(7):e2202751. doi:10.1002/adhm.202202751
112. Ji S, Zhu Z, Sun X, Fu X. Functional hair follicle regeneration: an updated review. Signal Transduct Target Ther. 2021;6(1):66. doi:10.1038/s41392-020-00441-y
113. Ma Y, Lin Z, Chen X, et al. Human hair follicle-derived mesenchymal stem cells promote tendon repair in a rabbit Achilles tendinopathy model. Chin Med J (Engl). 2023;136(9):1089–1097. doi:10.1097/CM9.0000000000002542
114. Yang H, Zhang Y, Du Z, Wu T, Yang C. Hair follicle mesenchymal stem cell exosomal lncRNA H19 inhibited NLRP3 pyroptosis to promote diabetic mouse skin wound healing. Aging (Albany NY). 2023;15(3):791–809. doi:10.18632/aging.204513
115. Huang YZ, He T, Cui J, et al. Urine-Derived Stem Cells for Regenerative Medicine: basic Biology, Applications, and Challenges. Tissue Eng - Part B Rev. 2022;28(5):978–994. doi:10.1089/ten.teb.2021.0142
116. Müller H, Hu J, Popp R, et al. Deleted in malignant brain tumors 1 is present in the vascular extracellular matrix and promotes angiogenesis. Arterioscler Thromb Vasc Biol. 2012;32(2):442–448. doi:10.1161/ATVBAHA.111.239830
117. Chen CY, Rao SS, Ren L, et al. Exosomal DMBT1 from human urine-derived stem cells facilitates diabetic wound repair by promoting angiogenesis. Theranostics. 2018;8(6):1607–1623. doi:10.7150/thno.22958
118. Kong Y, Shao Y, Ren C, Yang G. Endometrial stem/progenitor cells and their roles in immunity, clinical application, and endometriosis. Stem Cell Res Ther. 2021;12(1):474. doi:10.1186/s13287-021-02526-z
119. Dalirfardouei R, Jamialahmadi K, Jafarian AH, Mahdipour E. Promising effects of exosomes isolated from menstrual blood‐derived mesenchymal stem cell on wound‐healing process in diabetic mouse model. J Tissue Eng Regen Med. 2019;13(4):555–568. doi:10.1002/term.2799
120. Wang DR, Pan J. Extracellular vesicles: emerged as a promising strategy for regenerative medicine. World J Stem Cells. 2023;15(4):165–181. doi:10.4252/wjsc.v15.i4.165
121. Nallakumarasamy A, Jeyaraman M, Maffulli N, et al. Mesenchymal Stromal Cell-Derived Extracellular Vesicles in Wound Healing. Life. 2022;12(11):1733. doi:10.3390/life12111733
122. Xiong M, Zhang Q, Hu W, et al. The novel mechanisms and applications of exosomes in dermatology and cutaneous medical aesthetics. Pharmacol Res. 2021;166:105490. doi:10.1016/j.phrs.2021.105490
123. Théry C, Witwer KW, Aikawa E, et al. Minimal information for studies of extracellular vesicles 2018 (MISEV2018): a position statement of the International Society for Extracellular Vesicles and update of the MISEV2014 guidelines. J Extracell Vesicles. 2018;7(1):1535750. doi:10.1080/20013078.2018.1535750
124. Yu H, Wu Y, Zhang B, et al. Exosomes Derived from E2F1–/– adipose-Derived Stem Cells Promote Skin Wound Healing via miR-130b-5p/TGFBR3 Axis. Int J Nanomed. 2023;18:6275–6292. doi:10.2147/IJN.S431725
125. Yang D, Zhang W, Zhang H, et al. Progress, opportunity, and perspective on exosome isolation - Efforts for efficient exosome-based theranostics. Theranostics. 2020;10(8):3684–3707. doi:10.7150/thno.41580
126. Vyas KS, Kaufman J, Munavalli GS, Robertson K, Behfar A. Exosomes: the latest in regenerative aesthetics. Regener Med. 2023;18(2):181–194. doi:10.2217/rme-2022-0134
127. Buzas EI. The roles of extracellular vesicles in the immune system. Nat Rev Immunol. 2023;23(4):236–250. doi:10.1038/s41577-022-00763-8
128. Rybkowska P, Radoszkiewicz K, Kawalec M, et al. The Metabolic Changes between Monolayer (2D) and Three-Dimensional (3D) Culture Conditions in Human Mesenchymal Stem/Stromal Cells Derived from Adipose Tissue. Cells. 2023;12(1):178. doi:10.3390/cells12010178
129. Dong Y, S A, Rodrigues M, et al. Injectable and Tunable Gelatin Hydrogels Enhance Stem Cell Retention and Improve Cutaneous Wound Healing. Adv Funct Mater. 2017;27(24):1606619. doi:10.1002/adfm.201606619
130. Xu Q, Sigen A, Gao Y, et al. A hybrid injectable hydrogel from hyperbranched PEG macromer as a stem cell delivery and retention platform for diabetic wound healing. Acta Biomater. 2018;75:63–74. doi:10.1016/j.actbio.2018.05.039
131. Patra JK, Das G, Fraceto LF, et al. Nano based drug delivery systems: recent developments and future prospects. J Nanobiotechnology. 2018;16(1):71. doi:10.1186/s12951-018-0392-8
132. Fan Z, Jiang C, Wang Y, et al. Engineered extracellular vesicles as intelligent nanosystems for next-generation nanomedicine. Nanoscale Horiz. 2022;7(7):682–714. doi:10.1039/d2nh00070a
133. Wu P, Zhang B, Ocansey DKW, Xu W, Qian H. Extracellular vesicles: a bright star of nanomedicine. Biomaterials. 2021;269. doi:10.1016/j.biomaterials.2020.120467
134. Chen J, Zhao Q, Peng J, Yang X, Yu D, Zhao W. Antibacterial and mechanical properties of reduced graphene-silver nanoparticle nanocomposite modified glass ionomer cements. J Dent. 2020;96:103332. doi:10.1016/j.jdent.2020.103332
135. Xiao R, Ding J, Chen J, et al. Citric acid coated ultrasmall superparamagnetic iron oxide nanoparticles conjugated with lactoferrin for targeted negative MR imaging of glioma. J Biomater Appl. 2021;36(1):15–25. doi:10.1177/0885328220975570
136. Wang H, Ding L, Xu F, et al. Construction of novel amphiphilic chitosan-polylactide graft copolymer nanodroplets for contrast enhanced ultrasound tumor imaging. J Biomater Appl. 2021;36(4):613–625. doi:10.1177/08853282211011766
137. Gibello L, D’Antico S, Salafia M, et al. First pilot case-control interventional study using autologous extracellular vesicles to treat chronic venous ulcers unresponsive to conventional treatments. Pharmacol Res. 2023;190:106718. doi:10.1016/j.phrs.2023.106718
138. Yang T, Dong Y, Wan J, et al. Sustained Release of BMSC-EVs from 3D Printing Gel/HA/nHAP Scaffolds for Promoting Bone Regeneration in Diabetic Rats. Adv Healthc Mater. 2023;12(18):e2203131. doi:10.1002/adhm.202203131
© 2024 The Author(s). This work is published and licensed by Dove Medical Press Limited. The full terms of this license are available at https://www.dovepress.com/terms.php and incorporate the Creative Commons Attribution - Non Commercial (unported, v3.0) License.
By accessing the work you hereby accept the Terms. Non-commercial uses of the work are permitted without any further permission from Dove Medical Press Limited, provided the work is properly attributed. For permission for commercial use of this work, please see paragraphs 4.2 and 5 of our Terms.