Back to Journals » Biologics: Targets and Therapy » Volume 18
Aspergillus Species from the Sabkha Marsh: Potential Antimicrobial and Anticancer Agents Revealed Through Molecular and Pharmacological Analysis
Authors Sajer BH , Alshehri WA , Alghamdi SS , Suliman RS , Albejad A, Hakmi H
Received 6 April 2024
Accepted for publication 30 July 2024
Published 6 August 2024 Volume 2024:18 Pages 207—228
DOI https://doi.org/10.2147/BTT.S472491
Checked for plagiarism Yes
Review by Single anonymous peer review
Peer reviewer comments 3
Editor who approved publication: Professor Shein-Chung Chow
Bayan H Sajer,1,2 Wafa A Alshehri,3 Sahar S Alghamdi,4,5 Rasha S Suliman,6 Alhanouf Albejad,3 Haifa Hakmi3
1Biology Department, Faculty of Science, King Abdul Aziz University, Jeddah, Saudi Arabia; 2Immunology Unit, King Fahd Medical Research Centre, King Abdulaziz University, Jeddah, Saudi Arabia; 3Department of Biological Sciences, College of Science, University of Jeddah, Jeddah, 23890, Saudi Arabia; 4Department of Pharmaceutical Sciences, College of PharmacyKing Saud Bin Abdul Aziz University for Health Sciences (KSAU-HS), Riyadh, Saudi Arabia; 5King Abdullah International Medical Research Center, Riyadh, Saudi Arabia; 6Pharmacy department, Fatima College of health sciences, Abu Dhabi, United Arab Emirates
Correspondence: Bayan H Sajer, King Abdul Aziz University, P.O. Box 80200, Jeddah, 21589, Saudi Arabia, Email [email protected]
Introduction: This study aimed to investigate the fungal growth and diversity in the Sabkha marsh. The anti-bacterial properties of the isolated fungi were assessed using an agar disk diffusion assay, and the crude extracts were tested for their anticancer activities. Liquid chromatography-mass spectrometry was employed to identify the active compounds of the fungal secondary metabolites. In-silico studies were conducted to predict the toxicity, pharmacokinetic properties, and safety profiles of the identified compounds.
Results: The analysis revealed that the isolated fungi belonged to the Aspergillus species, specifically Aspergillus flavus and Aspergillus niger. The crude extract of A. flavus exhibited significant anticancer activity against various cancer cell lines, while the antifungal activities against pathogenic bacteria varied between the two fungi. Liquid chromatography-mass spectrometry analysis identified several compounds in the fungal isolates. In Aspergillus flavus, the compounds included Aflavinine, Dihydro-24-hydroxyaflavinine, Phomaligin A, Hydroxysydonic acid, Gregatin B, Pulvinulin A, Chrysogine, Aspergillic acid, Aflatoxin B1, and Aflatoxin G1. In Aspergillus niger, the compounds identified were atromentin, fonsecin B, firalenone, rubrofusarin, aurasperone E, aurasperone D, aurasperone C, nigerone, and αβ-dehydrocurvularin.
Conclusion: This study demonstrated promising fungal growth and diversity in the Sabkha marsh, with Aspergillus species being the most prevalent. The fungal crude extract showed anticancer activities against various cancer cell lines, while the antifungal activities against pathogenic bacteria varied between the two fungi. Future research should focus on investigating the antimicrobial activities of these fungi against multidrug-resistant bacteria and exploring the genetic changes in bacteria and cancer cells treated with these fungal extracts. Additionally, it is important to test the anticancer activity of the active compounds separately to determine which one is the active agent against cancer cells. This information can be used in drug development trials.
Keywords: saline environment, biological activity, Aspergillus genus, microbial isolation, antimicrobial screening
Graphical Abstract:
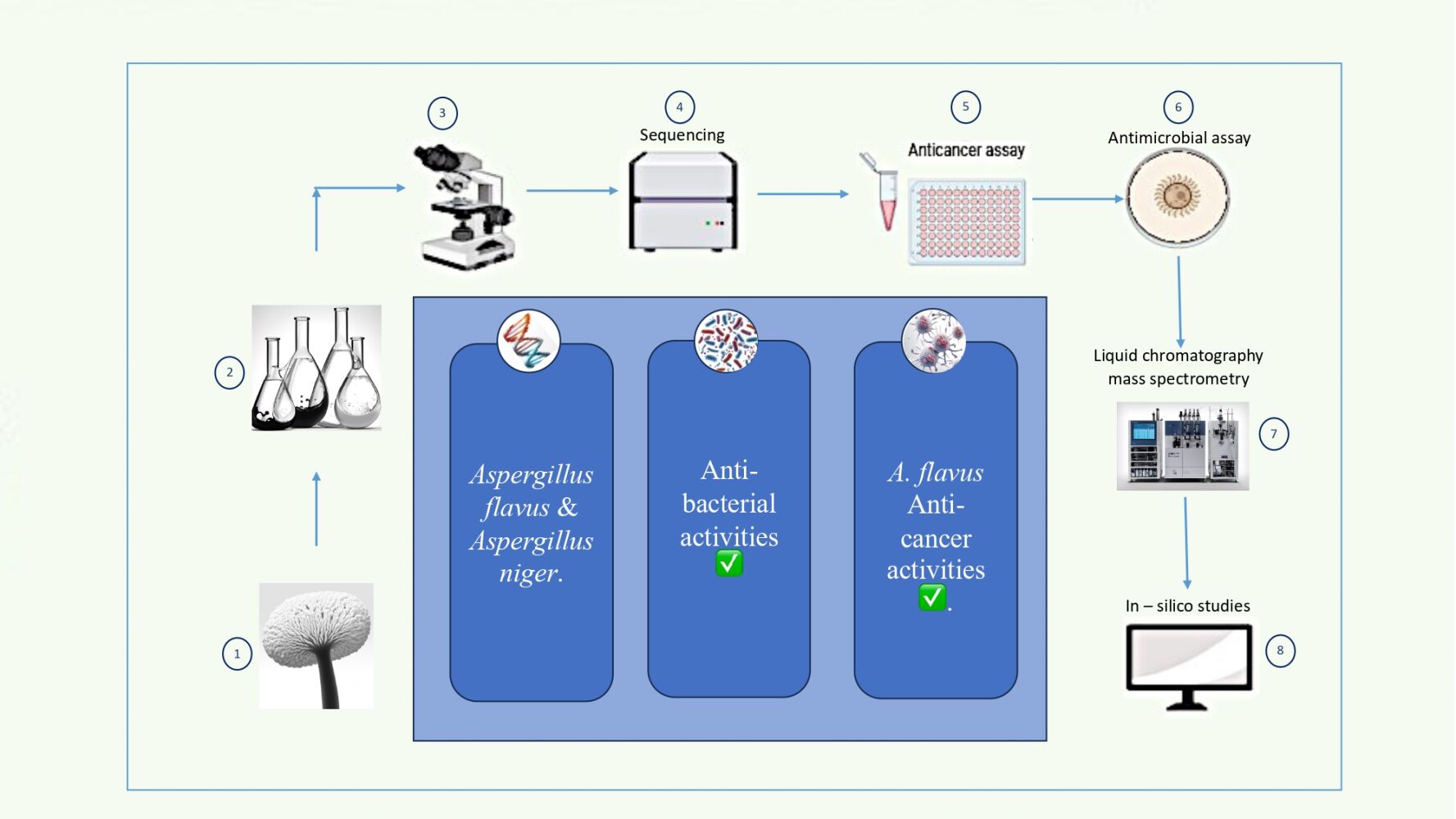
Introduction
The Sabkha desert sites in Saudi Arabia boast extreme environmental conditions, characterized by high salinity, neutral pH, and fluctuating moisture content, rendering them seemingly inhospitable for many life forms.1 Despite these challenges, recent studies have unveiled the remarkable resilience of a diverse range of fungi in the Sabkha environment, showcasing their ability to thrive under extreme environmental pressures.1
The presence of fungal species in the Sabkha desert not only underscores their capacity to adapt and flourish in challenging conditions but also sheds light on their pivotal role in maintaining ecological balance within extreme ecosystems.2 The diverse microbial communities thriving in these environments, including fungal species such as Aspergillus, Penicillium, Fusarium, and Chaetomium, demonstrate the fungi’s ability to endure harsh conditions and contribute to the ecological dynamics of the Sabkha desert.2
These findings underscore the necessity for further research into the adaptations and ecological significance of fungi in the Sabkha desert.2 Fungi in the Sabkha Desert have evolved unique biochemical pathways and specialized structures that enable them to thrive in extreme temperatures and arid conditions.3 They utilize mechanisms such as heat-shock proteins and compatible solutes to safeguard their cellular structures from damage caused by heat or cold. Furthermore, some desert fungi can enter a dormant state during extreme conditions, ensuring their survival until more favorable conditions prevail.4
Thermophilic fungi have garnered attention for their potential applications in biotechnology and medicine. Certain compounds produced by these fungi have exhibited promising antimicrobial properties, positioning them as potential candidates for the development of novel antibiotics.5
The intriguing anti-cancer properties of these compounds have catalyzed the exploration of novel therapeutic strategies. Researchers are delving into the use of these fungal enzymes as potential targets for drug development, aiming to harness their anti-cancer effects in a more targeted and controlled manner. By comprehending the mechanisms of action of these enzymes, scientists aim to unlock new possibilities for cancer treatment.
For instance, Aspergillus niger has been extensively studied, with previous research testing biogenic silver nanoparticles produced from the extract of Aspergillus niger as antimicrobial, anti-cancerous, and anti-angiogenic agents.6 Similarly, Ethyl Acetate Extracted from Aspergillus niger has demonstrated both anticancer and anti-microbial activities in a previous study.7 As for Aspergillus flavus, a study utilized it to produce selenium nanoparticles and conducted further testing to evaluate their potential for biological applications, including antiviral, anticancer, antibacterial, antioxidant, and antibiofilm properties.8 Additionally, a pyranone derivative obtained from Aspergillus flavus was previously tested for its antimicrobial and antitumor activities.9
The relationship between cancer and microorganisms, particularly fungi, has been extensively explored, with a growing emphasis on the potential of thermophilic fungi in biotechnology and medicine.5 However, there is still a research gap in fully exploring the potential of the active compounds derived from these fungi, which possess both anti-cancer and antimicrobial properties.10,11 This study marks the commencement of future research endeavors aimed at evaluating and testing these active compounds separately for their pharmaceutical potential. The objective is to highlight the potential of locally sourced natural compounds, derived from our own land, for pharmaceutical applications.
Materials and Methods
Isolation and Morphological Characterization of Fungi
To investigate the microflora in the region of Makkah, specifically Rabigh city in Saudi Arabia, thirty samples of Sabkha soil were collected. Soil samples (n = 5) were obtained from depths of 5 to 20 cm, placed in clean plastic bags, and transferred to the laboratory for examination. The collected samples were then prepared for fungi isolation using dilution and blotter techniques. Subsequently, they were incubated at a temperature of 25 ± 2 °C for 7 days.12
Pure colonies were stored on potato dextrose agar (PDA) slants at 4 °C for future use. The isolated colonies were examined for species identification using traditional methods. These methods involved characterizing their texture on specific media, including Sabouraud dextrose agar and Czapek-Dox agar, all supplemented with chloramphenicol. Furthermore, surface, reverse colony, and microscopic observations were performed.12
Physicochemical Analysis of Soil Samples
The soil samples were examined using a digital camera to classify particle characteristics, including color, shape, and morphology. pH analysis was conducted using a pH meter (model HI98107), and the moisture content (MC) was determined according to.13,14 Additionally, the electrical conductivity (EC) of each soil sample was measured using an EC meter (Mettler Toledo-AG).
Microscopic Examination of Isolates
In this investigation, we examined the external characteristics of colonies and assessed their colors to identify the fungi. We used scanning electron microscopy (SEM) to observe fungal biofilms on the crystalline surface of the substrate. Sample preparation included fixing, drying, and gold coating the samples. Finally, we analyzed the samples using a SEM (JEOL 7500FA JEOL, Peabody, MA, USA) at 10 kV.
Characterization at the Molecular Level of the Isolated Samples
Preparation of Mycelium and DNA Extraction
To prepare the mycelium, a 2 μL aliquot of potato dextrose broth (PDB) from Himedia, Mumbai, India was added to Potato Dextrose Agar (PDA) tubes and vortexed to disperse the spores. The spore-PDB mixtures were then added to flasks containing 100 mL of PDB and kept undisturbed at room temperature for two to three days. The mycelium was harvested by filtration, frozen at −80 °C for 30 minutes, lyophilized, and stored at −80 °C. Subsequently, the mycelium was ground in liquid nitrogen with a sterile mortar to obtain mycelium powder.
For DNA extraction, 20 mg of mycelium powder was used with a DNeasy Plant Mini Kit. The DNA quantity and quality were assessed by electrophoresis on a 0.8% agarose gel and visualized with ethidium bromide under UV transillumination.15
Amplification of ITS Region and PCR
The internal transcribed spacer (ITS) region of ribosomal DNA was amplified by PCR using the ITS1-F (5’-CTTGGTCATTTAGAGGAAGTAA-3’) and ITS4-R (5’-TCCTCCGCTTATTGATATGC-3’) primers. PCR amplification was carried out in a final volume of 50 μL. The amplified product was checked by electrophoresis on a 1% agarose gel and visualized with ethidium bromide under UV transillumination, following the manufacturer’s instructions.16
Sequencing and Analysis
The PCR product was purified using an Exo SAPIT kit, followed by Sanger sequencing at Beijing Genomic Institute (BGI), Hong Kong, China. The sequence was compared to the NCBI database using BLAST software. The obtained sequences were then aligned in Ugene using the T-Coffee algorithm.17 A phylogenetic tree was created using iTOL, an interactive tree tool, which offers various functionalities such as data upload, sharing projects, help resources, subscription information, and search capabilities.18
Antimicrobial Assay
The antibacterial properties of the fungal isolates were tested using an agar disk diffusion assay. Each fungal isolate was cultured on Sabouraud dextrose agar with 1% chloramphenicol for seven days at 28 °C. Afterward, 15 mm diameter disks were cut from the agar and placed on media plates inoculated with bacteria (blood agar, nutrient agar, and Sabouraud dextrose agar). The plates were then incubated at 37 °C for seven days. The antimicrobial activity was assessed by visualizing and measuring the inhibition zones (in mm).
Statistical Analysis
The data is presented as the mean plus or minus the standard deviation (SD) of three replicates.
Examining the Anticancer Activity
Preparing the Fungal Crude Extract
The contents of three fully grown fungal plates were transferred into a 500-mL beaker using a spatula under a biohazard cabinet, and 300 mL of brine solution was added to the beaker. The mixture was homogenized for 3–5 minutes at a rate of 7000–9000 rpm using the Ultra-Turrax® homogenizer. The homogenized mixture was then transferred into a specially designed 500-mL separating funnel see (Figure S1) in the Supplementary files and 300 mL of ethyl acetate was added. The mixture was stirred for 5 minutes at around 1100 rpm using an overhead stirrer, and the stirring was then stopped to allow the two layers to separate. The lower aqueous layer was transferred into a 500-mL conical flask, and the organic layer was drawn into another 500-mL conical flask with anhydrous sodium sulphate added to absorb the water. The aqueous phase was transferred back into the funnel, and the entire process was repeated twice. The organic phases were combined and filtered on paper into a 500-mL round bottom flask, then concentrated to a few mL on a rotary evaporator before being transferred into a tared vial. The crude extract was dried using a vacuum concentrator, and the vial was weighed and stored at 4°C until further use.
Cell Culture
In this study, six different cell lines were utilized. The hepatoma cell line HepG2, colorectal cancer cell lines HCT8 and HCT116, and the human drug-resistant metastatic breast cancer cell line MDA-MB-231 were procured from American Type Culture Collection (ATCC), United States. The epithelial-like breast carcinoma cell lines KAIMRC119 and triple-negative KAIMRC220 were obtained and characterized at King Abdullah International Medical Research Center (KAIMRC) in Riyadh, Saudi Arabia. All these cell lines were cultivated in advanced Dulbecco’s Modified Eagle Medium (DMEM) supplemented with 10% fetal bovine serum, 1% L-glutamine, and 1% antibiotics (Pen/Strep). The cells were cultured for a minimum of 24 hours before any experimentation, in 96-well plates at a density of 10,000 cells per well.
Cell Proliferation Assay (MTT)
In this study, the extracts from Aspergillus flavus isolate, and Aspergillus niger were investigated for their potential anticancer effects on the mentioned cell lines. The cells were exposed to varying concentrations of each extract, ranging from 0 to 1000 µg/mL. Doxorubicin, a chemotherapy drug, and mitoxantrone, an antineoplastic agent, were used as positive controls for assessing cell toxicity, while cells treated with Dimethylsulfoxide (DMSO) only were used as a negative control. After 48 hours, the cells were treated with 5 mg/mL MTT solution. Following a 3-hours incubation at 37 °C, the media was removed, and 100 µL of DMSO solvent was added to each well. After shaking the plate on an orbital shaker for 45 minutes, the absorbance was measured at OD 590 nm. The half-maximal inhibitory concentration (IC50) values for each substance were determined using the dose‒response curve in GraphPad Prism 8. Each experiment was repeated three times for accuracy.
Utilizing Liquid Chromatography–Mass Spectrometry (QTOF-LCMS) for Secondary Metabolite Identification
Aspergillus Flavus
The ethanolic extract of Aspergillus flavus was analyzed using total ion current spectra (TIC) raw data and the Mass Hunter qualitative and quantitative analysis software. The mass screening of the spectrum (Figure 1) yielded the following findings. Chemical characteristics were extracted from the LC‒MS data using the Molecular Features Extraction (MFE) algorithm and the recursive analysis workflow. These features were screened based on detected nodes at various retention times per minute, with a minimum intensity of 6000 counts, and aligned with previously detected compounds, taking into account adducts ([M+H]+ and [M-H]-).
Aspergillus Niger
The ethanolic extract of Aspergillus niger was analyzed using the Mass Hunter qualitative and quantitative analysis software, based on the total ion current spectra (TIC) raw data refer to (Figure 2). Following the mass screening of the spectrum (Figure 2), the following observations were made. Chemical characteristics were identified from the LC‒MS data using the Molecular Features Extraction (MFE) algorithm and the recursive analysis workflow. These features were obtained by evaluating the detected nodes at different retention times per minute, with a minimum intensity of 6000 counts, and were matched with previously identified compounds, taking into consideration adducts ([M+H]+ and [M-H]-).
Computational Predictions and Investigations
Computational Predictions for Secondary Metabolites
The secondary metabolites of the fungal samples were identified using a library of 28 chemical structures. These structures were then used in computational predictions to assess the biological anticancer activity, pharmacokinetic properties, and safety profiles of each molecule. The in silico predictions were performed using Simplified Molecular Input Line Entry System (SMILES) representations, which are summarized in Supplementary Table S1. The chemical structures of all the metabolites are shown in (Figures 3 and 4) for reference.
![]() |
Figure 3 The chemical Structures of Secondary Metabolites Identified in Aspergillus flavus. |
![]() |
Figure 4 The chemical Structures of Secondary Metabolites Identified in Aspergillus Niger. |
Utilization of PASS Online Web Server for Anticancer Activity Predictions
To predict the anticancer potential of the identified metabolites, we inputted the 2D chemical structures into the PASS Online web server (Prediction of Activity Spectra for Substances). This web server can predict over 4000 types of biological activity, including pharmacological effects, mechanisms of action, toxic and adverse effects, and more. The results are presented as a probability score (Pa), where a score exceeding 0.7 indicates strong anti-cancer properties. A score between 0.7 and 0.5 suggests moderate anti-cancer activity if experimentally tested.21
Predicting Pharmacokinetic Parameters and Oral Bioavailability
To assess various pharmacokinetic parameters for the identified metabolites, we utilized the SwissADME online web tool. These parameters include lipophilicity, molecular weight, solubility, insolubility, insaturation, and flexibility. The goal was to determine the likelihood of the metabolite being orally active. The results were presented using a radar plot, where the properties falling within the colored zone indicate the acceptable range for orally active drugs.22
Leveraging the PASS Online Web Server for Anticancer Activity Predictions
To predict the anticancer activity of the identified metabolites, we submitted their 2D chemical structures to the PASS Online web server. The results are presented as a probability score (Pa). A score exceeding 0.7 indicates robust anticancer properties, while a score between 0.7 and 0.5 suggests moderate anticancer activity if experimentally tested. The PASS Online web server predicts over 4000 types of biological activity, including pharmacological effects, mechanisms of action, toxic and adverse effects, and more. This provides valuable insights into the potential anticancer properties of the compounds.23
Assessment of Blockage of hERG K+ Channels
The potential cardiotoxicity of the identified metabolites was evaluated using the Pred-hERG 5.0 web server. This web server predicts the blockage of hERG K+ channels and provides probability predictions for each molecule, distinguishing between blocker and non-blocker. The Pred-hERG 5.0 web server utilizes advanced machine learning models and an extensive database to enhance accuracy and reliability in predicting hERG blockage. This assessment contributes to evaluating the cardiotoxicity of the compounds (https://tox-new.charite.de/protox_II/).24
Results
Soil Physicochemical Investigations
The soil samples collected from all locations showed a slightly alkaline pH. The electrical conductivity (EC) of the soil samples was highest at 82400 µS/CM. The soil texture was clay. Upon closer examination of the soil granules, variations in particle size and shape were observed among the different sites studied (see Table 1 and Figure 5).
![]() |
Table 1 Soil Characteristics and Microbial Abundance |
![]() |
Figure 5 Images of the study area of the Makkah region, Rabigh city (Sabkha). |
Macroscopic Results of Isolates
Conidial morphology-based characterization is a fundamental approach in fungal taxonomy.25 Scanning Electron Microscopy (SEM) offers a precise method for examining conidial ornamentation, allowing for a more distinct differentiation between various types of ornamentation. Given that both isolates belonged to the Aspergillus genus, the resemblance of their conidia was clearly evident under SEM (Figure 6).
![]() |
Figure 6 SEM images showing characteristic features of Aspergillus conidia: line (A) Aspergillus flavus, line (B) Aspergillus Niger. |
Molecular Identification of Fungal Strains
The strain was identified as Aspergillus flavus with a 100% match in both identity and coverage through the BLAST analysis. This strain has been assigned the accession number OR262346.1 and has been designated as WIS. The phylogenetic tree revealed the presence of three distinct clusters (Figure 7). Within one of these clusters, the Aspergillus flavus strain WIS was grouped alongside forty-two other A. flavus strains. Furthermore, it is noteworthy that the closest eight clusters also included strains of A. flavus.
![]() |
Figure 7 Phylogenetic tree showing the position of Aspergillus flavus strain WIS (acc. no. OR262346.1) and the closest related strains based on (ITS) region sequences. |
The final WIS isolate was confirmed as Aspergillus niger with a 100% match in both identity and coverage through BLAST analysis. This specific strain was labeled WIS and allocated the accession number OR262345.1. In the phylogenetic tree, three distinct clusters were evident, as shown in (Figure 8). Within one of these clusters, the Aspergillus niger strain WIS was grouped alongside thirty-six other A. niger strains. Notably, the closest cluster also encompassed several A. niger strains.
![]() |
Figure 8 Phylogenetic tree showing the position of Aspergillus Niger strain WIS (acc. no. OR262345.1) and the closest related strains based on (ITS) region sequences. |
Antimicrobial Assay
The antibacterial activities against pathogenic bacteria are presented in (Table 2) and (Figures 9 and 10).
![]() |
Table 2 Antibacterial Activity Using Fungi Aspergillus Flavus and Aspergillus niger Against Pathogenic Bacteria Evaluated by the Inhibition Zone Diameter in Mm |
For Aspergillus niger, the largest observed inhibition zone on blood agar was 11 mm, while the smallest inhibition zone on nutrient agar was 10.6 mm.
As for Aspergillus flavus, it only displayed an inhibition zone of 13.7 mm on blood agar.
Notably, both Aspergillus flavus and Aspergillus niger exhibited similar activity, with an inhibition zone of 14.8 mm, also on nutrient agar.
It should be emphasized that neither Aspergillus flavus nor Aspergillus niger had any effect on either the Gram-Positive bacteria S. aureus or the gram-negative bacteria E. coli on Sabouraud dextrose agar.
Evaluation of Anticancer Potential of Extracts on Various Cell Lines
Cells were exposed to a range of drug concentrations, ranging from 0 to 1000 µg/mL, to evaluate the potential anticancer properties of the extracts. The MTT assay was used to assess cell viability after a 48-hours incubation period. To measure growth inhibition, mitoxantrone and doxorubicin were employed as positive controls. We utilized six different cell lines, including breast cancer, hepatic cancer, and colorectal cancer, to diversify our findings. These cell lines have distinct genomic and proteomic profiles. Additionally, we used newly developed Saudi-specific KAIMRC1, ER+/PR+/HER2-, and KAIMRC2 triple-negative cell lines to target the local population.
The extracts from Aspergillus flavus isolate demonstrated significant anticancer effects on all cell lines, with IC50 values below 50 µg/mL. The IC50 results are summarized in (Table 3), and visual representations are provided in (Figure 11).
![]() |
Table 3 The IC50 (µg/Ml) Values of Different Extracts Against Six Cancer Cell Lines Using the MTT Cell Viability Assay |
Identification of Secondary Metabolites Using QTOF-LCMS
LCMS of Aspergillus Flavus
The tentatively identified compounds are Aflavinine,26 Dihydro-24-hydroxyaflavinine,26 Phomaligin A,27 Hydroxysydonic acid,28 Gregatin B,29 Pulvinulin A,29 Chrysogine,30 Aspergillic acid,30 Aflatoxin B1,31 and Aflatoxin G1.31 The mean m/z implies the measured m/z (Figure 1) (Table 4).
![]() |
Table 4 Compounds (M1–M10) Tentatively Identified from Aspergillus Flavus Extract |
LCMS Aspergillus Niger
The tentatively identified compounds are atromentin,32 fonsecin B,33 firalenone,34 rubrofusarin,33 aurasperone E,35 aurasperone D,35 aurasperone C,35 nigerone,36 and αβ-dehydrocurvularin.37 The mean m/z implies the measured m/z (Figure 2) (Table 5).
![]() |
Table 5 Compounds (M1–M10) Tentatively Identified from Aspergillus niger Extract |
Results for in-silico Predictions
Predicting Anti-Cancer Activities
The anti-cancer activity of the identified metabolites was evaluated using the PassOnline web server, a robust tool for predicting the potential anti-cancer properties of compounds. This analysis was conducted to determine the therapeutic advantages of the identified metabolites and their potential for the development of new anti-cancer drugs sourced from natural origins. The results, outlined in (Table 6), indicated that Aspergillus Flavus, along with two metabolites, Gregatin B and Pulvinulin A, exhibited promising anti-cancer activity, with Pa values of 0.902 and 0.880, respectively. Importantly, all the identified metabolites in Aspergillus niger demonstrated Pa values ranging from 0.709 to 0.854, suggesting that this fungus could be a potential reservoir for discovering highly effective anti-cancer agents.
![]() |
Table 6 The Predicted Probabilities of Anti-Cancer Activity for the Identified Metabolites in Aspergillus Flavus, and Aspergillus niger Fungi Samples |
Evaluation of Pharmacokinetic Parameters
In this section, we showcase the results derived from using the SwissADME online web server, a potent tool for evaluating the pharmacokinetic characteristics of the identified metabolites. Through this thorough analysis, we successfully evaluated diverse pharmacokinetic parameters and ascertained the potential oral activity of the metabolites.
In (Figure 12), the results of this analysis are presented, indicating that most of the metabolites demonstrate pharmacokinetic properties within the recommended range for lipophilicity, molecular weight, solubility, insolubility, insaturation, and flexibility. Notable metabolites showing favorable properties include Phomaligin A, Hydroxysydonic acid, Gregatin B, Pulvinulin A, Aspergillic acid, Aflatoxin B1, Aflatoxin G1, Fonsecin B, and αβ-dehydrocurvularin. However, some of the remaining metabolites exhibit deviations in one or more parameters. Further analysis and refinement may be necessary to optimize their pharmacokinetic properties for potential use as anti-cancer agents. These findings provide valuable insights into the development of new orally active anti-cancer drugs derived from natural compounds.
![]() |
Figure 12 Pharmacokinetic Properties for the Identified Metabolites Using SwissADME Webserver. |
Assessment of Metabolite Toxicity for Anti-Cancer Applications
An extensive evaluation was carried out to assess the toxicity of the identified metabolites to ensure their safety and suitability for anti-cancer applications. The results of the comprehensive toxicity assessment are summarized in (Table 7). Chrysogine, among the analyzed metabolites, demonstrated a predicted hepatotoxicity of 50%. Additionally, three metabolites - Aflatoxin B1, Aflatoxin G1, and αβ-dehydrocurvularin - were determined to possess a carcinogenicity of 60%. Immunotoxicity analysis revealed significant activity in 12 metabolites, with a probability range from 0.52 to 0.99. Moreover, only 5 metabolites exhibited mutagenic potential, while none were found to be cytotoxic. These findings offer valuable insights into the safety profile of the identified metabolites for further development as anti-cancer agents.
![]() |
Table 7 Comprehensive Toxicity Assessment of the Identified Metabolites Using Protox II Webserver |
Prediction of Cardiac Toxicity
In order to conduct a thorough assessment of the potential toxicity of the identified metabolites, it was essential to investigate their ability to block the hERG channel, a known cause of cardiac toxicity. Alongside the evaluation of organ and endpoint toxicity, a cardiac toxicity assessment was performed using the pred-hERG web server. This analysis aimed to determine the metabolites’ capacity to inhibit the hERG cardiac potassium channel and potentially induce adverse cardiovascular effects. Notably, the majority of the metabolites displayed no indications of cardiac toxicity (refer to Table 8), with the exception of Aflavinine and Aflatoxin G1, which exhibited a 62.81% and 52.47% probability of blocking the hERG channel, respectively.
![]() |
Table 8 Evaluation of Cardiac Toxicity in the Identified Metabolites Using the Pred-hERG Webserver |
Discussion
The two species identified belonged to the Aspergillus genus, known for their xerophilic nature, thriving in limited water availability.38 Notably, the antibacterial activity of Aspergillus flavus and Aspergillus niger against E. coli underscores their potential as sources of new antibiotics. Furthermore, the varying degrees of antibacterial activity against pathogenic bacteria and the media-dependent nature of this activity open avenues for the development of novel antifungal agents.
In addition to their antibacterial properties, the extracts of Aspergillus flavus and Aspergillus niger have shown promising antifungal activity against various cancer cell lines. The potent efficacy of these extracts against aggressive and triple-negative breast cancer cell lines highlights their potential in the development of new anti-cancer drugs.20,39 Further research into the cytotoxic effects of these extracts at the protein level will provide valuable insights into the underlying mechanisms and pathways involved in their anti-cancer activity.40
Moreover, the mass spectrometry analysis revealed the identification of several parent compounds, shedding light on the chemical composition of the samples studied. These compounds, such as fonsecin B, rubrofusarin, and aurasperone, hold promise for the discovery of new bioactive molecules with potential pharmaceutical applications in cancer and antibiotic drug development.33–37,41
Furthermore, the comprehensive evaluation of the identified metabolites provides crucial insights into their toxicity profiles, emphasizing the need for further research to understand their toxicological implications. This study lays the foundation for the development of new orally active anticancer drugs derived from natural compounds and informs risk assessment and mitigation strategies for potential health risks associated with these metabolites.42,43
The potential of these findings in the discovery of new anti-cancer and antibiotic drugs, coupled with the importance of continued research to address toxicity implications, positions this study at the forefront of future drug development and risk assessment in the field of cancer therapeutics and antimicrobial agents.
Conclusion
In conclusion, the study highlights the potential of metabolites derived from thermophilic fungi as a source of novel anti-bacterial and anti-cancer agents. The significant biological activity and favorable pharmacokinetic properties of the crude extract of these thermophilic fungi are promising. Certain metabolites indicate their potential for further development, so they should be studied separately. However, further optimization and refinement are necessary to overcome limitations and enhance their efficacy as antibiotics and anti-cancer drugs.
Overall, this study contributes to the ongoing efforts in the discovery and development of effective antibiotics and anti-cancer therapies from natural compounds.
Disclosure
The authors report no conflicts of interest in this work.
References
1. Alotaibi MO, Sonbol HS, Alwakeel SS, et al. Microbial diversity of some sabkha and desert sites in Saudi Arabia. Saudi J Biol Sci. 2020;27(10):2778–2789. doi:10.1016/j.sjbs.2020.06.038
2. Al Disi ZA, Naja K, Rajendran S, et al. Variability of blue carbon storage in arid evaporitic environment of two coastal Sabkhas or mudflats. Sci Rep. 2023;13(1). doi:10.1038/s41598-023-39762-7
3. Gostinčar C, Grube M, Gunde-Cimerman N. Evolution of fungal pathogens in domestic environments? Fungal Biol. 2011;115(10):1008–1018. doi:10.1016/j.funbio.2011.03.004
4. Gostinčar C, Lenassi M, Gunde-Cimerman N, Plemenitaš A. Fungal Adaptation to Extremely High Salt Concentrations. Adv Appl Microbiol. 2011;77:71–96. doi:10.1016/B978-0-12-387044-5.00003-0
5. Orfali R, Perveen S. New bioactive metabolites from the thermophilic fungus penicillium sp. isolated from ghamiqa hot spring in Saudi Arabia. J Chem. 2019;2019:1–7. doi:10.1155/2019/7162948
6. Pasha A, Kumbhakar DV, Sana SS, et al. Role of biosynthesized Ag-NPs using aspergillus niger (MK503444.1) in antimicrobial, anti-cancer and anti-angiogenic activities. Front Pharmacol. 2022;12. doi:10.3389/fphar.2021.812474
7. Niazi SK, Basavarajappa DS, Kumaraswamy SH, et al. GC-MS based characterization, antibacterial, antifungal and anti-oncogenic activity of ethyl acetate extract of aspergillus niger strain AK-6 isolated from rhizospheric soil. Curr Issues Mol Biol. 2023;45(5):3733–3756. doi:10.3390/cimb45050241
8. Mohammed EJ, Abdelaziz AEM, Mekky AE, et al. Nanoparticles: a Green Synthesis Approach to Antiviral, Anticancer, Anti-Biofilm, and Antibacterial Applications. Pharmaceuticals. 2024;17(7):915. doi:10.3390/ph17070915
9. Elaasser MM, Abdel-Aziz M. Antioxidant, antimicrobial, antiviral and antitumor activities of pyranone derivative obtained from Aspergillus candidus; 2011. Available from: www.scholarsresearchlibrary.com.
10. Ghfar AA, El-Metwally M, Shaaban M, et al. Production of terretonin N and butyrolactone I by thermophilic aspergillus terreus TM8 promoted apoptosis and cell death in human prostate and ovarian cancer cells. Molecules. 2021;26(9):2816. doi:10.3390/molecules26092816
11. Sajer BH, Alshehri WA, Alghamdi SS, Suliman R. Extract of microbial isolates from the western region of the kingdom of Saudi Arabia and their applications in the field of health. J Contemp Med Sci. 9(6):420–430.
12. Frisvad JC, Samson RA, “Polyphasic taxonomy of Penicillium subgenus Penicillium A guide to identification of food and air-borne terverticillate Penicillia and their mycotoxins”. Available from: www.cbs.knaw.nl/.
13. Cruz-Romero M, Smiddy M, Hill C, Kerry JP, Kelly AL. Effects of high pressure treatment on physicochemical characteristics of fresh oysters (Crassostrea gigas). Innovative Food Sci Emerg Technol. 2004;5(2):161–169. doi:10.1016/j.ifset.2004.01.002
14. Jackson M. Organic matter determination for soils. Soil Chemical Analysis; 1958.
15. Bruns T, Gardes M. Molecular tools for the identification of ectomycorrhizal fungi—taxon-specific oligonucleotide probes for suilloid fungi. Mol Ecol. 1993;2(4):233–242. doi:10.1111/j.1365-294X.1993.tb00013.x
16. White TJ, Bruns T, Lee S, Taylor J. Amplification and direct sequencing of fungal ribosomal RNA genes for phylogenetics. PCR Protocols. 1990;18:315–322.
17. Okonechnikov K, Golosova O, Fursov M, Ugene T. Unipro UGENE: a unified bioinformatics toolkit. Bioinformatics. 2012;28(8):1166–1167. doi:10.1093/bioinformatics/bts091
18. Letunic I, Bork P. Interactive Tree Of Life v2: online annotation and display of phylogenetic trees made easy. Nucleic Acids Res. 2011;39(suppl):W475–W478. doi:10.1093/nar/gkr201
19. Ali R, Samman N, Al Zahrani H, et al. Isolation and characterization of a new naturally immortalized human breast carcinoma cell line, KAIMRC1. BMC Cancer. 2017;17(1). doi:10.1186/s12885-017-3812-5
20. Ali R, Al Zahrani H, Barhoumi T, et al. Isolation and establishment of a highly proliferative, cancer stem cell-like, and naturally immortalized triple-negative breast cancer cell line, kaimrc2. Cells. 2021;10(6):1303. doi:10.3390/cells10061303
21. Daina A, Michielin O, Zoete V. SwissADME: a free web tool to evaluate pharmacokinetics, drug-likeness and medicinal chemistry friendliness of small molecules. Sci Rep. 2017;7(1). doi:10.1038/srep42717
22. Drwal MN, Banerjee P, Dunkel M, Wettig MR, Preissner R. ProTox: a web server for the in silico prediction of rodent oral toxicity. Nucleic Acids Res. 2014;42(W1):W53–W58. doi:10.1093/nar/gku401
23. Braga RC. Tuning hERG out: antitarget QSAR Models for Drug Development HHS Public Access; 2014. Available from: http://labmol.farmacia.ufg.br/predherg.
24. Varga J, Due M, Frisvad JC, Samson RA. Taxonomic revision of Aspergillus section Clavati based on molecular, morphological and physiological data. Stud Mycol Centraalbureau voor Schimmelcult. 2007;59:89–106. doi:10.3114/sim.2007.59.11
25. Filimonov DA, Lagunin AA, Gloriozova TA, et al. Prediction of the Biological Activity Spectra of Organic Compounds Using the Pass Online Web Resource. Chem Heterocycl Compd. 2014;50(3):444–457. doi:10.1007/s10593-014-1496-1
26. Wang H-J, Gloer JB, Wicklow DT, Dowd PF. Aflavinines and Other Antiinsectan Metabolites from the Ascostromata of Eupenicillium crustaceum and Related Species. Appl Envir Microbiol. 1995;61(12):4429–4435. doi:10.1128/aem.61.12.4429-4435.1995
27. Evidente A, Cristinzio G, Punzo B, Andolfi A, Testa A, Melck D. Flufuran, an antifungal 3,5-disubstituted furan produced by Aspergillus flavus Link. Chem Biodivers. 2009;6(3):328–334. doi:10.1002/cbdv.200800292
28. Hamasaki T, Nagayama K, Hatsuda Y. Two new metabolites, sydonic acid and hydroxysydonic acid, from aspergillus sydowi. Agric Biol Chem. 1978;42(1):37–40. doi:10.1080/00021369.1978.10862919
29. Kithsiri Wijeratne EM, Xu Y, Arnold AE, Leslie Gunatilaka AA. Pulvinulin A, Graminin C, and cis-Gregatin B-New Natural Furanones from Pulvinula sp. 11120, a Fungal Endophyte of Cupressus arizonica; 2015. Available from: http://www.ebi.ac.uk/Tools/msa/muscle/.
30. Rank C, Klejnstrup ML, Petersen LM, et al. Comparative chemistry of Aspergillus oryzae (RIB40) and A. flavus (NRRL 3357). Metabolites. 2012;2(1):39–56. doi:10.3390/metabo2010039
31. Abbas HK. Aflatoxin and Food Safety. Crc Press; 2005.
32. Demarque DP, Crotti AEM, Vessecchi R, Lopes JLC, Lopes NP. Fragmentation reactions using electrospray ionization mass spectrometry: an important tool for the structural elucidation and characterization of synthetic and natural products. In: Natural Product Reports. Vol. 33. 3. Royal Society of Chemistry. 432–455. doi:10.1039/c5np00073d
33. Zhang Y, Ling S, Fang Y, Zhu T, Gu Q, Zhu W-M. Isolation, structure elucidation, and antimycobacterial properties of dimeric aphtho-γ-pyrones from the marine-derived fungus aspergillus carbonarius. Chem Biodivers. 2008;5(1):93–100. doi:10.1002/cbdv.200890017
34. Inokoshf J, Shiomib K, Masumab R, Tanakaa H, Yamadab H, Omura S. Funalenone, a Novel Collagenase Inhibitor Produced by Aspergillus Niger. J Antibiot. 1999;52(12):1095–1100.
35. Li DH, Han T, Guan L-P, et al. New naphthopyrones from marine-derived fungus Aspergillus Niger 2HL-M-8 and their in vitro antiproliferative activity. Nat Prod Res. 2016;30(10):1116–1122. doi:10.1080/14786419.2015.1043553
36. Song YC, Li H, Ye YH, Shan CY, Yang YM, Tan RX. Endophytic naphthopyrone metabolites are co-inhibitors of xanthine oxidase, SW1116 cell and some microbial growths. FEMS Microbiol Lett. 2004;241(1):67–72. doi:10.1016/j.femsle.2004.10.005
37. Xiang C, Liu Y, Liu S-M, et al. αβ-Dehydrocurvularin isolated from the fungus Aspergillus welwitschiae effectively inhibited the behaviour and development of the root-knot nematode Meloidogyne graminicola in rice roots. BMC Microbiol. 2020;20(1). doi:10.1186/s12866-020-01738-2
38. Vinnere Pettersson O, Leong SL. Fungal Xerophiles (Osmophiles). In: eLS. Wiley; 2011. doi:10.1002/9780470015902.a0000376.pub2
39. Huang Z, Yu P, Tang J. Characterization of triple-negative breast cancer MDA-MB-231 cell spheroid model. Onco Targets Ther. 2020;13:5395–5405. doi:10.2147/OTT.S249756
40. Kumari R, Jaiswal H, Bharti R, Jayachandran LE, Ghosh AK. Apoptotic Effects of Afl atoxin B1 Purifi ed from Aspergillus Flavus of Stored Wheat Grains in India on Human Cells. Adv J Toxicol Curr Res. 2020;4(1):29–037.
41. Xiao JF, Zhou B, Ressom HW. Metabolite identification and quantitation in LC-MS/MS-based metabolomics. TrAC Trends Anal Chem. 2012;32:1–14. doi:10.1016/J.TRAC.2011.08.009
42. Lipinski CA. Lead- and drug-like compounds: the rule-of-five revolution. Drug Discover Today. 2004;1(4):337–341. doi:10.1016/j.ddtec.2004.11.007
43. Lipinski CA, Lombardo F, Dominy BW, Feeney PJ. Experimental and computational approaches to estimate solubility and permeability in drug discovery and development settings. Adv Drug Deliv Rev. 1997;23(1–3):3–25. doi:10.1016/S0169-409X(96)00423-1
© 2024 The Author(s). This work is published and licensed by Dove Medical Press Limited. The
full terms of this license are available at https://www.dovepress.com/terms.php
and incorporate the Creative Commons Attribution
- Non Commercial (unported, v3.0) License.
By accessing the work you hereby accept the Terms. Non-commercial uses of the work are permitted
without any further permission from Dove Medical Press Limited, provided the work is properly
attributed. For permission for commercial use of this work, please see paragraphs 4.2 and 5 of our Terms.