Back to Journals » Nanotechnology, Science and Applications » Volume 17
Antibacterial, Antibiofilm, and Tooth Color Preservation Capacity of Magnesium Oxide Nanoparticles Varnish (in vitro Study)
Authors Hamalaw SJ , Kareem FA , Noori AJ
Received 11 March 2024
Accepted for publication 14 June 2024
Published 27 June 2024 Volume 2024:17 Pages 127—146
DOI https://doi.org/10.2147/NSA.S462771
Checked for plagiarism Yes
Review by Single anonymous peer review
Peer reviewer comments 2
Editor who approved publication: Professor Kattesh Katti
Sonya Jamal Hamalaw, Fadil Abdulla Kareem, Arass Jalal Noori
Department of Pedodontics and Community Oral Health, College of Dentistry, University of Sulaimani, Sulaymaniyah, Iraq
Correspondence: Sonya Jamal Hamalaw, Sulaimaniyah, 46001, Iraq, Tel +9647705049108, Email [email protected]
Purpose: Antibacterial and antibiofilm properties of magnesium oxide nanoparticles (MgONPs) mixture assessed against Streptococcus mutans (S. mutans), in addition to examining MgONPs varnish impact on the preservation of the tooth color and inhibition of methylene blue diffusion to the enamel.
Methods: MgONPs mixture was prepared in deionized water (DW), absolute ethanol (E), and rosin with ethanol (RE), named varnish. The antibacterial and antibiofilm capacities of MgONPs mixtures were tested by agar well diffusion, colony-forming unit (CFU), and biofilm inhibition microtiter methods in triplicate and compared to sodium fluoride varnish (NaF) and chlorhexidine mouthwash (ChX). A spectrophotometer was used to record basic tooth color. The artificial demineralization was initiated for 96 h. Then, experimental materials were applied to the corresponding group, and 10-day pH cycles proceeded. Then, the color was recorded in the same ambient environment. The methylene blue diffusion was evaluated by staining the samples for 24 h. After that, the diffusion test was calculated by a digital camera attached to the stereomicroscope.
Results: The agar well diffusion test expressed a significant inhibition zone with all MgONPs mixtures (p = 0.000), and maximum inhibition zone diameter associated with MgONPs-RE. The same finding was observed in the CFU test. Additionally, 2.5%, 5%, and 10% MgONPs-RE varnish showed strong biofilm inhibition capacity (p = 0.039) compared to NaF and ChX groups that inhibit biofilm formation moderately (p = 0.003). The study shows that the 5% MgONPs-RE varnish maintains basic tooth color with minimal methylene blue diffusion compared to NaF varnish (p = 0.00).
Conclusion: Evaluating MgONPs as a mixture revealed antibacterial and antibiofilm capacity against S. mutans with a higher effect of MgONPs-RE varnish. Also, examining the topical effect of MgONPs-RE varnish on the preservation of the tooth color after pH cycle challenges and methylene blue diffusion to enamel confirmed the high performance of MgONPs-RE varnish at 5%.
Keywords: fluoride, chlorhexidine, methylene blue, rosin, biofilms, dental enamel
Graphical Abstract:
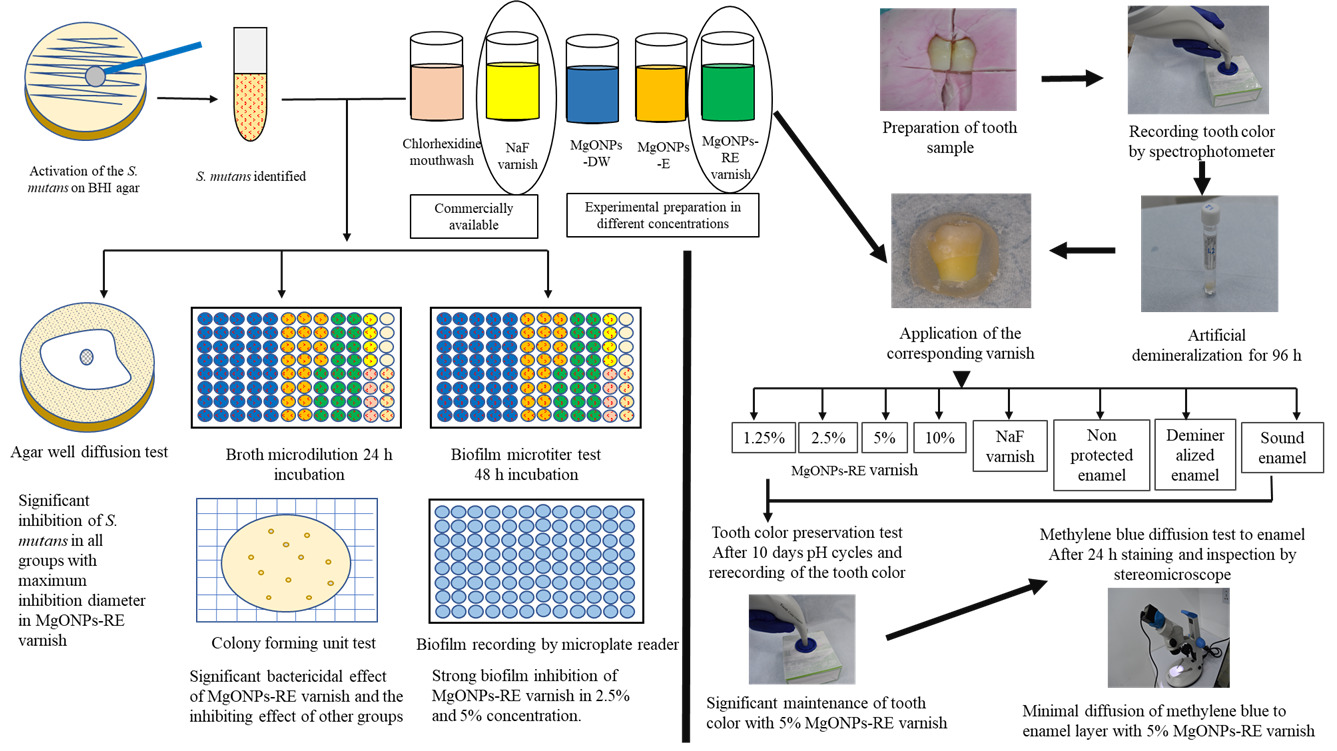
Introduction
S. mutans is classified as a member of the oral microbial community that efficiently uses dietary sucrose to synthesize major contributors of extracellular polysaccharide matrix in dental biofilms.1,2 Studies indicate that S. mutans isolates, which colonize the human oral cavity, are more capable of forming biofilms than isolates of other Streptococcus species.3 Compared to other planktonic microorganisms, those form biofilms have a low pH survival capacity.4 Additionally, S. mutans facilitates the attachment and survival of acidogenic and aciduric species by developing a rich extracellular polysaccharide and a low-pH environment to act synergistically to form dental caries.5
The first step of dental caries starts with demineralizing the exposed tooth surface, mostly enamel.6 Despite enamel’s chemical and mechanical importance, it provides a natural, harmonious color to the teeth. According to some, one of a person’s most crucial interactive communication talents is having an attractive smile,7 which is achieved by an acceptable dental arrangement that complements their lips, gingiva, and faces with the understanding of the reality of light and tooth color.8,9
The consequences of the demineralization lead to the initiation of white color lesions. According to definitions, a white spot lesion is subsurface enamel micro spaces from decay demineralization that manifests as a milky white porosity when distributed on smooth surfaces.6 The demineralized enamel showed different opacities than the normal enamel because of light reflection between air, water, and enamel. The light reflection index of enamel is 1.62. A watery substance with a 1.33 reflection index fills the holes of the subsurface lesion. Water and enamel have different refractive indices, causing an opaque lesion appearance.10 Drying surfaces replace water with air, which has a refractive index of 1 and lowers the transmission coefficient of enamel from 0.483 to 0.313 mm-1 at 525 nm wavelength of the light source, resulting in a larger refractive index and transmission coefficient difference between enamel and air.11 The outcome lesion is more noticeable, and an early lesion might be found.10
The familiar antimicrobial product that is applied in dentistry is ChX. Discriminatory ChX obstructs the gram-positive bacterial activity, including S. mutans.12 However, the antibacterial effect of the ChX product does not strengthen the tooth surface by remineralization as it is composed of identical bis-biguanide components of two chloroguani joined by a central hexamethylene chain13 and free from minerals. Many attempts have been applied to reinforce the enamel lesion by remineralizing minerals, first starting with fluoride,14 and the reprecipitation of the mineral lost with dental products containing fluoride is limited to the outermost layer without subsurface remineralization.15,16
Therefore, a dental product with tooth protection capacity is always a critical subject for investigation. Nonetheless, there is controversy regarding fluoride’s antibacterial properties, as it was shown to have a role in decreasing the glycolytic activities of S. mutans. Still, their role in clinical application is unclear.17,18
The dramatic effects of nanomaterials for biomedical and clinical applications have been thoroughly studied during the last few decades.19,20 Because of their novel and improved surface-to-volume ratio, distinctive mechanical and chemical properties, and higher cell bioavailability, many nanomaterials are used in medicine, pharmacology, dental supplies, and others. Magnesium’s (Mg) biocompatibility and physiological dissolving properties attract researchers to employ magnesium derivative as a biomaterial for medical uses,21,22 with safety declaration of Mg by the United States Food and Drug Administration.23
In addition, the researchers were inspired to examine the antibacterial effects of MgONPs against various bacterial isolates24–26 with the investigation that approves the protection capacity of enamel by MgONPs application.27 However, the lack of studies on the antibacterial and antibiofilm properties of MgONPs and their ability to protect the enamel color encourages the development of this study. Despite the justification for testing MgONPs, significant results were necessary to reject or accept the null hypothesis about the ineffectiveness of MgONPs. The present study analyzed the bacterial and biofilm inhibition impact of MgONPs in different mixtures and concentrations against most cariogenic bacteria (S. mutans) and compared them to commercially available ChX mouthwash and NaF varnish. Also, MgONPs’ capacity to preserve tooth color and strengthen enamel surface to inhibit methylene blue diffusion as an external stain was evaluated after initiation of artificial enamel lesion and pH cycling challenge.
Materials and Methods
Materials
MgONPs (MgO, 99.9%, 10–30 nm, SkySpring Nanomaterials, Houston, USA), absolute ethanol (CASNo: 64–17-5, Scharlab, Sentmenat, Spain), fully hydrogenated rosin (Foral AX-E, Code: P75041E1, Eastman Chemical Middelburg BV, Netherland), deionized water (Ref: M1975-500G, TS-ISO 3696, Macit and Macit, Turkey), Tryptone Yeast Extract Cystine Bacitracin (TYCSB) agar (Ref: M1975-500G, Himedia, Mumbai, India), Brain Heart Infusion (BHI) broth (Ref: 610008, Liofilchem, Roseto d. Abruzzi (TE), Italy), Brain Heart Infusion (BHI) agar (Ref: 610007, Liofilchem, Roseto d. Abruzzi (TE), Italy), Mueller Hinton (MH) agar (Ref: M173-500G, Himedia, Mumbai, India), 5% sodium fluoride varnish (NPN: 80022817, AMD Medicom, Canada, USA), chlorhexidine DG fluoride 0.12% mouth wash (KIN, Barcelona, Spain) and Polystyrene flat clear base cell Culture plate (Sorfa life science research) and Crystal violet (CAS: 548–62-9, Sas Biochem chemopharma, Cosne Sur Loire, France), potassium hydroxide (KOH) pellets (CAS No: 1310–58-3, Biochem chemopharma, France), calcium chloride anhydrous fused (CaCl2) (CAS No: 10043–52-4, Biochem chemopharma, France), potassium dihydrogen ortho phosphate anhydrous analytical reagent (KH2PO4) (CAS No: 7778–77-0, Biochem chemopharma, France), potassium chloride (KCl) (CAS No: 7447–40-7, Biochem chemopharma, France), acetic acid glacial analytical reagent (CAS No: 64–19-7, Biochem chemopharma, France), 2-(4-(2-hydroxyethyl)-1-piperazinyl) ethane sulfonic acid (HEPES) buffer (CAS No: 7365–45-9, Biochem chemopharma, France), methylene blue (Sinopharm chemical reagent, China).
Methods
Study Design
The study groups were demonstrated in two parts, the first part evaluated the antibacterial and antibiofilm effects of MgONPs as shown in Figure 1, and the second part related to the tooth color preservation capacity of MgONPs.
![]() |
Figure 1 Study design and groups related to the antibacterial and antibiofilm capacity of MgONPs. |
MgONPs Characterization
MgONPs were analyzed by X-ray diffraction (XRD) applying PAN analytical X’Pert PRO at 45 kV with a current of 40 mA (CuKα=1.5406 Å). The inspecting rate was 1°/min in the 2θ range from 5° to 75. MgONPs’ morphology was examined by scanning electron microscope (SEM, Quanta 450), and the chemical composition of MgONPs was analyzed by energy-dispersive X-ray spectroscopy (EDX) performed in SEM.
Experimental MgONPs Mixture Preparation
MgONPs were prepared with DW, absolute ethanol, and rosin in different concentrations, as illustrated in Table 1. Testing different solutions and concentrations was to demonstrate the MgONPs impact as a single formula and in combination with other materials to symbiotic its effect on the S. mutans. Ethanol was used as an apportioning liquid with MgONPs due to ethanol usage as the main dispenser in commercial and experimental varnish produced by other researchers.28–30 For 10 minutes, the MgONPs mixture was blended with an ultrasonic homogenizer (Ti horn, 20 kHz at 65% efficiency, Bandelin electronic, 12,207 Berlin, Germany). The agglomeration of the MgONPs mixture was diminished for the statistically approved duration determined by the Pradhan et al study.31 The 10% MgONPs produce difficulty in pipetting and manipulation because of agglomeration, especially in DW; the examination of 10% MgONPs-DW was not included in the study.
![]() |
Table 1 Experimental Mixture and Varnish Composition |
Microorganism
S. mutans Activation
S. mutans activation was obtained from a glycerol-cultured store of isolated S. mutans with National Library of Medicine web BLAST and an accession number (OQ656871) from GenBank of another study.27
Antibacterial Efficacy of MgONPs Mixture Against S. mutans Tests
Agar Well Diffusion
The agar well diffusion method, a modification of the Kirby–Bauer method, was used to determine the inhibition zone of the experimental mixtures and varnish against S. mutans.33,34 The stock solution of S. mutans was prepared by obtaining a colony from freshly cultured S. mutans and adjusted on 0.5 McFarland using normal saline 0.9%. A sterile 100 µL pipette tip was used to gently punch the newly prepared Mueller Hinton (MH) agar plates without tearing the agar surface. Following labeling the plates, the agar was smeared with swab after being immersed in the stock solution of the experimental bacteria in the biosafety cabinet, one swab being used for each plate. The MH agar plate was punched once or twice to control misreading between different inhibition zones and incorrect recording. Then, 40 µL of the corresponding MgONPs mixture with five wells for each concentration was dispensed by pipette into the predetermined wells. Also, NaF varnish and 0.12% ChX were used alongside the experimental groups as a control.
Moreover, the commercially available 5% NaF varnish was diluted with absolute ethanol in a 1:1 ratio to decrease the viscosity of the varnish and facilitate its manipulation with a 2.5% concentration. Then, the inoculated plates with the holding mixture and the varnish in the wells were incubated for 24 h in 5% CO2 at 37 °C. The inhibition zone mean was measured in millimeters along the three imaginary lines passing through the middle of each well. Consequently, the recorded data were used to compare the means and ± Std (Standard deviation) of MgONPs mixture impact on S. mutans growth.
Colony-Forming Unit (CFU)
Broth microdilution methods were applied with double-fold dilution and a few modifications.35 A solution of 0.5 McFarland of S. mutans was prepared and diluted 100-fold (1:100) in the BHI broth to acquire (1x106 CFU/mL). Then, 96 wells of sterile polystyrene with a clear, flat base were prepared by distributing 100 µL of the adjusted BHI broth with S. mutans over each well. This was followed by the use of 100 µL of each concentration to the corresponding well, starting from MgONPs-DW from 5% to 0.039%, including 8 concentrations to evaluate the concentration’s effect of the MgONPs on the S. mutans, MgONPs-E from 10% to 1.25%, MgONPs varnish from 10% to 1.25%, 2.5% NaF varnish, 0.12% ChX, positive and negative control showed in Figure 2. Each concentration was prepared separately and transferred to the corresponding well as a consequence of two-fold dilutions. After mixing the broth and the solution by pipetting 3 times into the well, they were placed in the incubator for 24 h in 5% CO2 at 37 °C.33,36 Because of the high turbidity of the MgONPs in each well, the optical density (OD) could not be obtained accurately; therefore, CFU was applied to evaluate the S. mutans growth.
![]() |
Figure 2 Diagram showing colony-forming unit test (CFU) steps. |
The bactericidal concentration was evaluated by colony counting through dilution of the incubated microdiluted plate of the previous plate in 1:100 fold in the normal saline 0.9% and inoculation of 100 µL of the same dilution on the freshly prepared BHI agar.37 Subsequently, the inoculum was spread over the agar using sterile glass L-shaped spreaders, as presented in Figure 2. The inoculated BHI plates were placed in the incubator for 48 h in 5% CO2 at 37 °C. Triple BHI agar plates were inoculated for each concentration to calculate the value of the colony counted in this assay. A colony count between 30 and 300 was considered an inhibitory concentration. Less than 30 was considered bactericidal, and over 300 was considered uncountable.38
Biofilm Inhibitory Capacity by Microtiter
With minor modifications, a 96-well polystyrene microtiter flat and clear plate was used to analyze the anti-biofilm impact of the MgONPs mixture as applied by Mohanta et al.39 The S. mutans stock solution was adjusted in 0.5 McFarland in BHI broth. Into each well of the plates was poured 180 µL of BHI broth and 10 µL of the S. mutans stock solution. Then, 10 µL of each of the separately prepared two-fold concentrations, MgONPs-DW from 5% to 0.0039%, MgONPs-E from 10% to 1.25%, MgONPs-RE from 10% to 1.25%, 2.5% NaF varnish, 0.12% ChX, positive and negative control, were dispensed into the corresponding wells of 4 wells for control and 5 wells for experimental groups. The mixture was pipetted 3 times to ensure proper mixing. Another 96-well plate without bacteria was prepared using the same method to adjust the OD of the mixture. The plates were placed in the incubator for 48 h in 5% CO2 at 37 °C. The assessed time for biofilm formation by the Streptococcus group of bacteria was estimated to be from 12 h to 120 h.40 Following incubation, the microtiter plate well contents were disposed of, and the wells were gently cleaned with phosphate-buffered saline (PBS, pH 7.2) to eliminate non-adherent bacterial cells. The microtiter plates’ wells were then air-dried for 45 min. Following drying, adhering “sessile” bacteria in the wells were fixed with sodium acetate at a concentration of 2% w/v. The wells were stained with crystal violet dye of 0.1% w/v and left in a dark, isolated place for 30 min. The extra color was then properly removed by washing the wells with sterile DW. After each well had dried completely, 200 µL of 99% ethanol was added, and the OD at 630 Hz was measured by a microplate reader (BioTek Instrument, Inc., Highland Park, Winooski, VT 05404–0998, USA). The classification of the biofilm inhibition capacity was based on other research41 with modifications expressed in Table 2. All concentrations were calculated with this formula:
OD630 of biofilm inhibition capacity per well = (OD630 of tested concentration/OD630 of the blank of same concentration).
![]() |
Table 2 Optical Density of 630 Used to Classify the Film Inhibitory Impact of the MgONPs Mixture and Varnish |
Tooth Color Preservation Test
This test was performed to evaluate the MgONPs-RE varnish in 4 concentrations, which were 1.25%, 2.5%, 5%, and 10% and comparing 8 groups, including (1.25%, 2.5%, 5%, 10%) MgONPs-RE varnish and 5% NaF varnish, non-protected enamel, demineralized enamel, and sound enamel group with randomly placing 10 samples per each group. The non-protected enamel group underwent both artificial demineralization and 10-day pH cycles, while the demineralized group was only subjected to artificial dimerization for 96 h, as shown in Figure 3.
![]() |
Figure 3 Study design and groups included to evaluate tooth color preservation capacity of MgONPs. |
Tooth Sample Preparation
The first upper bicuspid teeth extracted for orthodontic treatment were cleaned from debris and blood and then stored in deionized water, which was replaced every week until required. A slow-moving handpiece was used to polish the teeth (Geared angle handpiece, Foshan COXO Medical Instrument, China, model-CX235C1) with rubber polishing cups (TPC, INC 851 S. Lawson ST, CA 91748, USA) and pumice (30-micron, PD, Switzerland) to eliminate stains and biological debris from the tooth’s surface. Every tooth was inspected under a stereomicroscope to rule out teeth with demineralization, cracks, and dental caries. Each tooth root was dissected with a cutting disk using a slow-moving micromotor, and then the tooth was separated mesiodistally. With transparent cold cure acrylic, the enamel portion was secured to a 20 mL fecal specimen container spatula to mould teeth sections of the same size. The same container was prepared in a way that is compatible with the tip of the portable spectrophotometer by using silicon impression material with the negative impression of the spatula holding the tooth section. The reference point of the spatula in the container held all samples in the same reference position during the test, as expressed in Figure 4. After that, each sample was detached from the spatula and used for the test.
![]() |
Figure 4 Diagrammatic figure of a photograph illustrating steps of tooth color measurement and methylene blue diffusion test. |
Tooth Color Measurement
The color of the tooth section was measured before and after the application of the treatments by a portable spectrophotometer specialized for tooth color recording (Tooth color comparator, Aries Outlets, B08PHPBHQ5). The tooth color measurement in this study reflects the measurement of three variables: value, hue, and chroma. The value measures the brightness or darkness of the tooth color assessed independently of the hue. Hue indicates the predominant color of the teeth. Chroma is the characteristic that defines the degree of purity of the hue.42 The comparator was set at a single spot measurement with a Vita classical shad guide coded with (V 29). Before the measurements, the spectrophotometer was calibrated using a manufacturer-standards calibrator and instructions. Evaluating tooth color by a spectrophotometer is considered a reliable and more reproducible method than visual observation of the color.43 The color was recorded in the same environment in the prepared box to eliminate external effects.44
Each sample was immersed in deionized water and then placed over the absorbent tissue directly before the measurement of the color. The difference in the colors was obtained by recording the same sample’s color before and after using the varnishes following the completion of the pH cycles for 10 days. For statistical evaluation, the letter corresponding to the hue part of the color was coded as (M = 1, L = 2, and R = 3), and the value and chroma part of the color were already recorded in numbers that could be statistically calculated.
De-Remineralization Solution Preparation
In this study, demineralization solution was prepared by using the formula 2.2 mM CaCl2, 2.2 mM NaH2PO4, 0.05 M acetic acid, and KOH (1 M) to adjust pH to 4.4.45 In the in vitro studies, various remineralization solutions were used to have identical properties to natural saliva; however, selection of the remineralization solution that did not interfere with the remineralization effect of the experimental solution had a reliable effect on the result. Therefore, the remineralization solution prepared in this study did not depend on the solution containing fluoride. The ingredients of the remineralization solution were 1.5 mM CaCl2 × 2 H2O, 0.9 mM KH2PO4, 150 mM KCl, 20 mM HEPES. The remineralization’s solution pH was adjusted to 7.0 using KOH.46
Artificial Demineralization
Each sample was retained in the 10 mL demineralization solution for 96 h in the incubator at 37%, with the solution changed daily.46,47 After completion of the demineralization cycle, the samples were carried out from the solution and prepared for the application of the experimental varnish.
Application of Experimental Varnish
Consequently, 10 µL of the corresponding varnish was painted to the enamel portions and dried for a minute. Then, the teeth were exposed to ionic exchange for 4 h in 20 mL of DW.48 The last group, known as the “sound enamel group”, comprised healthy teeth segments that were included without any protection or treatment to verify the accuracy of the color measurement before and after the cycles.
Re-Demineralization Cycle Application
The samples were placed in the 10 mL remineralization solution for 20 h during the day and over the night in the incubator at 37%. Later, a demineralization solution was used to complete the cycle by placing the sample separately in the 10 mL test tube for 4 hours. The re-demineralization cycles were continued for 10 days. Between the application of each solution, the samples were washed with 10 mL of deionized water to purity the tooth samples.
Methylene Blue Diffusion Test to Enamel
The histological examination of the tooth samples was evaluated with the benefit of Prabhakar’s research idea with the modification.49 The same tooth samples used for the color measurement were immersed in 2% methylene blue dye solution individually for 24 h at room temperature without agitation. After that, the samples were removed from the solution and washed with tap water for one minute. The samples were dried at room temperature on the tissue paper without touching the surface of the enamel and photographed by stereomicroscope. Then, the samples were sectioned longitudinally from the occlusal to the cervical part into two sections with the diamond disc.
The sections were evaluated under a stereomicroscope to determine the dye penetration, as shown in Figure 4.
Penetration of the dye calibrated by the S. viewer program specialized with the microscope camera (Llutico, UHD-5M-AAC, SN: 210831012, China) attached to a stereomicroscope (Optika, SN: 449994, Italy). Each section was measured for the enamel thickness, and 4 different penetration depths of the dye were recorded to determine the representative mean of the penetration per sample. The exposure target and time were set at 128, with 50% magnification and white balance setting for each group. All images and measurements of the sections were obtained at a stereomicroscope magnification of 40. Methylene diffusion depth per sample was calculated to unify the effect of diffusion of methylene dye as follows:
Methylene diffusion depth of sample = (mean methylene diffusion depth of sample × Enamel thickness of sample)/mean enamel thickness of all samples.
Statistics
Normally distributed data were calculated by the analysis of variance (ANOVA) with the Tukey post hoc test (p < 0.05) as in the agar well diffusion test. The Kruskal Wallis H (one-way ANOVA on ranks) test with paired multiple comparisons measured the non-normally distributed data in the CFU and biofilm inhibition tests. Median and Wilcoxon tests were applied for the paired data relating to the tooth color measurement. Mean and one-way ANOVA tests were applied for the parametric data belonging to the methylene blue penetration. The data were presented in a table and bar chart for each test using SPSS version 25.
Results
MgONPs Characterization
The MgONPs were in white powder form with fine particles. The XRD showed perfect identification of MgONPs diffraction peaks by applying the data to Match (3.15 build 252) with the entry number (96–900-0506) in Figure 5A. The SEM showed clustering of the MgONPs in Figure 5B. The EDX revealed an atomic percentage of 51.909% of magnesium (Mg) and 48.09% of oxygen (O) concentration in Figure 5C, representing highly pure MgONPs composition.
![]() |
Figure 5 Characterization tests of MgONPs (A) XRD, (B) SEM, (C) EDX.. |
Antibacterial Efficacy of MgONPs Mixture Against S. mutans Tests
Agar Well Diffusion
This assay revealed normal distribution. The inhibition zones of all groups express a clear inhibition zone with significant results. The results obtained by ANOVA analysis were MgONPs-DW (F(4, 24) = 286.077, p = 0.000), MgONPs-E (F(5,29) = 252.656, p = 0.000) and MgONPs-RE varnish (F(5,29) = 15.407, p = 0.000). The higher the amount of the MgONPs in all groups, the larger the inhibition zone diameter. However, the results revealed that the synergistic effect of the 10%, 5%, and 2.5% MgONPs-RE varnish inhibition zone diameters were larger than that of ChX (control group) and around twice that of the NaF varnish inhibition zone in Figure 6. The absolute ethanol alone inherited a shadow of less than 2 mm encircling the well. At the same time, MgONPs-E illustrated higher inhibition of S. mutans growth but was lower than ChX and had a similar effect to NaF varnish. However, the MgONPs-DW exhibited a lower antibacterial effect than the ChX mouthwash, with the highest inhibition at 5%.
Colony-Forming Unit (CFU)
The triplicate CFU testing of each mixture concentration indicated non-normally distributed values with significant differences between MgONPs-DW and MgONPs-RE varnish. Decreased CFU of S. mutans growth was observed in MgONPs-DW, similar to ChX in the 1.25% and decreased CFU with increasing concentrations, with H (8)=16.783, p = 0.032, while a slightly lower effect than in ChX was seen in the (0.625% to 0.078%). The 5% and 2.5% synergistic effect of MgONPs-RE varnish revealed a significantly greater decrease in the CFU of S. mutans than the other groups, with H (5)=11.683, p = 0.039. However, MgONPs-E exhibited a non-significant effect despite the comparable reduction in the S. mutans growth, with p = 0.055. A CFU score lower than 30 was considered a bactericidal effect, and that was the case for all MgONPs-RE varnish concentrations and the 5% of MgONPs-DW.
Biofilm Inhibitory Capacity by Microtiter
The nonnormally distributed data were analyzed by the Kruskal–Wallis H-test, followed by pairwise comparisons, and interpreted according to the weak, moderate, or strong biofilm inhibitory capacity classification. All MgONPs mixtures significantly inhibited biofilm production by S. mutans. Interpretation of the values recorded by the MgONPs-DW groups revealed that H(10)=29.284, p = 0.001, MgONPs-E groups H(7)=21.002, p = 0.004 and MgONPs-RE varnish groups H(7)=21.269, p = 0.003. Consequently, 2.5%, 5%, and 10% MgONPs-RE varnish showed strong biofilm inhibition capacity. Also, the NaF varnish and ChX groups showed moderate biofilm inhibitory capacity against S. mutans, as shown in Figure 7.
![]() |
Figure 7 Bar chart graph of MgONPs’ biofilm inhibition capacity by microtiter test on the S. mutans in the different concentrations and mixtures (MgONPs-DW, MgONPs-E and MgONPs-RE). |
Tooth Color Preservation Test
A Wilcoxon matched-pairs signed rank test was applied to determine the difference between the sound tooth’s color components before using any procedure and after applying the experimental varnish and passing through the pH cycles. The results of that analysis indicated a significant variation in the tooth color for each value z = −3.522; p = 0.000 and hue z = −3.464; p = 0.001. Meanwhile, the color variation was not significant for the tooth’s chroma component.
In the end, the median differences between the experimental groups reveal that 1.25%, 2.5%, 5%, and 10% MgONPs varnish maintains the tooth color near to its basic normal color in Figure 8. On the other hand, 5% NaF varnish had a higher value, but the hue remains nearly the same as its basic tooth color. Non-protected enamel was a group that expressed the largest value and chroma record after the pH cycle in contrast to the other experimental groups and inhibited the darker color of the tested tooth.
Methylene Blue Diffusion Test to Enamel
A one-way ANOVA test was used to identify the effect of experimental varnish on preventing methylene blue diffusion to the enamel. The test disclosed that there was a statically significant difference in the prevention of methylene blue diffusion to enamel in the groups that were protected by 1.25%, 2.5%, 5%, and 10% MgONPs varnish and 5% NaF varnish compared to non-protected enamel (F (7–32) = [106.428], p = 0.00) presented in Figure 9.
![]() |
Figure 9 Line chart showing mean of methylene blue diffusion to enamel calibrated by stereomicroscope with the digital camera. |
Post Hoc Tukey’s HSD test for multiple comparisons between groups expresses that the mean value of methylene blue diffusion was significantly different between 1.25%, 2.5% MgONPs varnish, and 5% NaF varnish (p = 0.00, 95% CI = [0.023, 0.126], p = 0.02, 95% CI = [0.004, 0.107]). Additionally, the lowest mean diffusion of the methylene blue of 5% MgONPs varnish compared to other MgONPs varnish concentrations and non-protected enamel groups (p = 0.00).
Despite the difference in means, 5% NaF varnish statistically was not different from 5% MgONPs varnish (p = 0.463), 10% MgONPs varnish (p = 0.248), and demineralized enamel (p = 0.967) in methylene blue diffusion depth. Histologically, diffusion of the methylene blue to enamel was less than 150 µm in the 5% MgONPs, followed by demineralized teeth and 5% NaF varnish in Figure 10C, G and E. The other groups of the MgONPs varnish, including 1.25%, 2.5%, and 10% in Figure 10A, B and D, showed decreasing in diffusion of methylene blue to enamel but comparably less than 5% MgONPs and 5% NaF varnish. The non-protected enamel group reveals the deepest diffusion of the methylene to the enamel, approximately 400 µm, compared to sound enamel, which expresses almost zero diffusion in Figure 10F and H.
Discussion
Topical fluoride application has been usually used for remineralizing the tooth layers for a long time;14 however, its antibacterial capacity has not been clarified.18,50 Additionally, the toxicity of fluoride overdose could form systemic complications,51 especially in high caries-risk individuals treated with multiple fluoride supplements. The US Food and Drug Administration encouraged the use of magnesium oxide (MgO) in biomedical applications and first approved its use in 1985 (21CFR184.1431).52
In this research, MgONPs mixtures were prepared in different concentrations with DW to evaluate their antibacterial and antibiofilm activity as an inherited property of MgONPs. The other two groups of MgONPs were designed with ethanol and a combination of rosin and ethanol in higher concentrations to analyze their potential effectiveness as a topical dental product for the last mixture. As previously investigated, nanoparticle impact was modified by the amount and contact time.53 Evaluating relatively high concentration of MgONPs with ethanol and rosin justified by the purpose of using MgONPs as dental varnish, which is the most effective, controllable form with a small amount of the topical dental treatment according to the American Dental Association (ADA) Council on Scientific Affairs.54
MgONPs in the various mixtures exhibit antibacterial and antibiofilm impact against S. mutans with the most prominent synergistic MgONPs-RE varnish. The same result was confirmed by other researchers who investigated the MgONPs’ antibacterial effect against S. mutans; however, they used MgONPs in different compositions and applications. For instance, they incorporated MgONPs with glass ionomer and applied them for dental restoration24 or coated them with zein for proposed use in a dental product.55 Another article reported that antibacterial efficacy against six oral microorganisms, including S. mutans, was improved by adding MgONPs.56
MgONPs are known for their antibacterial properties, but antibacterial mechanism actions were interpreted differently. Some theories reported that increased pH and Mg2+ ions might contribute to the antimicrobial processes, especially with higher concentrations, because disassociation of MgONPs produces OH− ions and Mg2+ ions.57 Reactive oxygen species (ROS) formation by MgONPs is another antibacterial mechanism of activity demonstrated by this nanoparticle, which causes bacterial lipid peroxidation and microbial death.58 Nguyen et al found that MgONPs could bind to the bacterial cell surface and cause distraction. However, the binding of nanoparticles affects the gram-negative bacteria more than the gram-positive bacteria, which is associated with differences in the cell membrane. Despite that, even the entrapment of the MgONPs on the bacteria membrane may affect the bacteria’s binding, communication, and overall virulency.57 The combined action could explain the enhanced effect of MgONPs’ antibacterial capacity against S. mutans in this study. The microdilution test showed inhibition of the S. mutans. In contrast, the CFU test confirmed the same finding with the highest inhibition of the S. mutans colonies. Still, the growth was not eliminated, which could illustrate the last mechanism of action by paralyzing the S. mutans virulency.
The most mineralized tissue in the human body is dental enamel.59 Even though enamel is distracted when it encounters environmental challenges and exposure to cariogenic bacteria. Early identification of caries allows nonsurgical therapy60 to remineralize the tooth surface to increase tooth tissue preservation and prevent the patient from beginning a lengthy cycle of restoration throughout life.61 There are several therapeutic approaches at various clinical levels of enamel lesions. A dental expert may initially suggest or apply topical supplements, reduce sugar consumption, or use toothpaste with an age-appropriate dose of fluoride.62 Even though maintaining excellent oral hygiene has never been simpler, despite advancements in oral care products, the incidence of dental is continuously rising.63 This is related to the multifactorial characteristic of dental caries, which is composed of the interaction of the host, diet, oral bacteria, and time.61,64–66
Besides the growing amount of oral care products, demands concentrate on preserving and improving tooth color in addition to periodontal disease and caries prevention.67 Almost always, prevention is better than cure. The findings of this study statistically support the protection of the enamel structure after demineralization with the treatment of the MgONPs-RE varnish in various amounts as a mixture that had efficient antibacterial and antibiofilm activity. The idea was to 1) apply the mineral that could reprecipitate and occupy the mineral diffused out from the enamel in the demineralization cycle, 2) through the remineralization approach seal further loss of the enamel minerals when it is exposed to re-demineralization cycles, 3) examine the intimacy of the enamel surface by measuring basic refractive indices of the tooth samples by spectrophotometer, which was measure value, hue and chroma before and after the pH cycles.
The groups expressing minimum change in the tooth color were statistically considered more preservative and healable for the enamel lesion as it nearly equilibrates the light component reflex with the basic tooth color. Five percent MgONPs varnish statistically showed the smallest unfavorable variation in the tooth color that was less than the 5% NaF varnish, with the tendency to preserve the tooth color in the other MgONPs groups, as illustrated in Figure 9. This could be associated with the infiltration of the MgONPs from the experimental varnish to the enamel surface as its diffusion and inhibition of mineral dissolving impact had been proved for the sound enamel in the previous investigation.27
Approximately the same finding was observed statistically in the methylene blue diffusion test. Both MgONPs and NaF varnish possess a barrier on the enamel surface to inhibit the diffusion of the dye into the enamel crystal, and the difference in the diffusion depth was obvious when comparing them to the non-protected enamel group in Figures 9 and 10. Furthermore, tooth enamel protected by 5% MgONPs varnish exhibits minimum diffusion of the methylene blue compared to the other groups. This could be the result of optimum remineralization of the MgONPs on the demineralized enamel surface, which sealed the microscopic porosity produced by the low pH cycle and formation of the smaller crystal size as it is clarified in other researches.30,68
On the other hand, a 5% NaF varnish impact was manifested to produce darker tooth shad than MgONPs varnish groups. This could be explained by the fluoride’s superficial effect on the enamel’s outer surface only and the varnish’s high rosin content. The outer surface enamel layer formation protects the tooth from further demineralization but at the same time prevents infiltration of the other essential ions deep into the tooth,69 and the variation in the tooth color remains as it is affected by the demineralization process in the subsurface area. In contrast, fluoride’s potential on the tooth color was not investigated in the literature; mostly, the impact on the restoration color was evaluated, and the result was acceptable.70,71 In another research, by observation, fluoride varnish was considered unpreferable as it causes transient discoloration of the teeth assessed by patients and dental hygienist.72
Nonetheless, the MgONPs varnish effect on tooth color preservation was supported by Abdulla et al’s research, which revealed Mg re-precipitation and a whiter appearance of the tooth color.68 The unavailability of the investigation on the MgONP effect on tooth color produces difficulty in comparing the statistical findings of this experiment to others. The obvious limitation of this study was that the exact clinical application and environment could not be duplicated because it is an in vitro study. The combination of antimicrobial activity, enamel protective capacity, and preservation of the tooth color are ideal demands necessary in dental products. This study on the topical impact of MgONPs on tooth color and prevention of methylene blue diffusion to enamel, besides their antibacterial and antibiofilm capacity, occupies the first position in the current experiment in the literature. Also, the present study’s finding provokes a sparkle for evaluating MgONPs varnish biocompatibility and clinically for targeting dental prevention and esthetical requirement of the teeth color in the high dental caries individuals.
Conclusion
The study concludes that MgONPs’ antibacterial capacity against S. mutans, a well-known cariogenic bacterium, has been demonstrated in all mixtures, even in low concentrations, by agar well diffusion and CFU tests. However, the most significant effects of the antibacterial and antibiofilm mixture were exhibited by 2.5 and 5% MgONPs-RE varnish. The antibacterial inhibition impact of the MgONPs-RE varnish in the higher concentrations was comparably more effective than in NaF and ChX. The antibiofilm properties of MgONPs-RE varnish concentrations were classified as strongly inhibiting S. mutans biofilm in the concentrations of 2.5%, 5%, and 10%.
Both experimental MgONPs-RE and NaF varnish demonstrate significant tooth color maintenance and methylene blue diffusion inhibition into the enamel. Furthermore, 5% MgONPs-RE varnish expressed a minimum change in the tooth color after completion of the pH cycles; this finding was also coincident with the higher prevention of the methylene blue diffusion to the enamel.
Ethics Approval and Informed Consent
This study was allowed by the Ethics Committee of the College of Dentistry, University of Sulaimani (No. 23/166). A tooth bank is absent in the current area, and the upper first bicuspid teeth extracted for orthodontic purposes were included in this study. Therefore, informed consent was not applicable, although the patients approved using their extracted teeth for research purposes.
Data Sharing Statement
The data supporting this study’s results are available from the corresponding author upon reasonable request.
Acknowledgments
The authors appreciate the cooperation of the colleagues and staff of the College of Dentistry at the University of Sulaimani.
Author Contributions
All authors made a significant contribution to the work reported, whether that is in the conception, study design, execution, acquisition of data, analysis, and interpretation, or in all these areas; took part in drafting, revising, or critically reviewing the article; gave final approval of the version to be published; have agreed on the journal to which the article has been submitted; and agree to be accountable for all aspects of the work.
Disclosure
The authors report no conflicts of interest in this study.
References
1. Koo H, Xiao J, Klein MI, Jeon JG. Exopolysaccharides produced by Streptococcus mutans glucosyltransferases modulate the establishment of microcolonies within multispecies biofilms. J Bacteriol. 2010;192(12):3024–3032. doi:10.1128/JB.01649-09
2. Leme AFP, Koo H, Bellato CM, Bedi G, Cury JA. The role of sucrose in cariogenic dental biofilm formation—new insight. J Dent Res. 2006;85(10):878–887. doi:10.1177/154405910608501002
3. Tamura S, Yonezawa H, Motegi M, et al. Inhibiting effects of Streptococcus salivarius on competence-stimulating peptide-dependent biofilm formation by Streptococcus mutans. Oral Microbiol Immunol. 2009;24(2):152–161. doi:10.1111/j.1399-302X.2008.00489.x
4. Krzyściak W, Jurczak A, Kościelniak D, Bystrowska B, Skalniak A. The virulence of Streptococcus mutans and the ability to form biofilms. Europ J Clin Microbiol Infect Dis. 2014;33(4):499–515. doi:10.1007/s10096-013-1993-7
5. Lemos J, Palmer S, Zeng L, et al. The Biology of Streptococcus mutans. Microbiol Spectr. 2019;7(1):10.1128/microbiolspec.GPP3-0051–2018. doi:10.1128/microbiolspec.GPP3-0051-2018
6. Bishara SE, Ostby AW. White spot lesions: formation, prevention, and treatment. Semin Orthodont. 2008;14(3):174–182. doi:10.1053/j.sodo.2008.03.002
7. Hattab FN, Qudeimat MA, al-Rimawi HS. Dental discoloration: an overview. J Esthet Dent. 1999;11(6):291–310. doi:10.1111/j.1708-8240.1999.tb00413.x
8. Mayekar SM. Shades of a color. Illusion or reality? Dent Clin North Am. 2001;45(1):155–172. doi:10.1016/S0011-8532(22)00474-8
9. Terry DA, Geller W, Tric O, Anderson MJ, Tourville M, Kobashigawa A. Anatomical form defines color: function, form, and aesthetics. Pract Proced Aesthet Dent. 2002;14(1):59–67.
10. Kidd EM, Fejerskov O. What constitutes dental caries? Histopathology of carious enamel and dentin related to the action of cariogenic biofilms. J Dent Res. 2004;83:C35–38. doi:10.1177/154405910408301s07
11. Brodbelt RHW, O’Brien WJ, Fan PL, Frazer-Dib JG, Yu R. Translucency of human dental enamel. J Dent Res. 1981;60(10):1749–1753. doi:10.1177/00220345810600100401
12. Evans A, Leishman S, Walsh L, Seow W. Inhibitory effects of antiseptic mouthrinses on Streptococcus mutans, Streptococcus sanguinis and Lactobacillus acidophilus. Austral Dental J. 2015;60(2):247–254. doi:10.1111/adj.12312
13. Gilbert P, Moore LE. Cationic antiseptics: diversity of action under a common epithet. J Appl Microbiol. 2005;99(4):703–715. doi:10.1111/j.1365-2672.2005.02664.x
14. Bawden JW. Fluoride varnish: a useful new tool for public health dentistry. J Public Health Dent. 1998;58(4):266–269. doi:10.1111/j.1752-7325.1998.tb03007.x
15. Tuloglu N, Bayrak S, Tunc ES, Ozer F. Effect of fluoride varnish with added casein phosphopeptide-amorphous calcium phosphate on the acid resistance of the primary enamel. BMC Oral Health. 2016;16(1):103. doi:10.1186/s12903-016-0299-4
16. Shen P, Bagheri R, Walker GD, et al. Effect of calcium phosphate addition to fluoride containing dental varnishes on enamel demineralization. Aust Dent J. 2016;61(3):357–365. doi:10.1111/adj.12385
17. Gupta A, Sharda S, Shafiq N, Kumar A, Goyal A. Topical fluoride-antibacterial agent combined therapy versus topical fluoride monotherapy in preventing dental caries: a systematic review and meta-analysis. Eur Arch Paediatr Dent. 2020;21(6):629–646. doi:10.1007/s40368-020-00561-7
18. Buzalaf MAR, Pessan JP, Honório HM, Cate ten V. Mechanisms of action of fluoride for caries control. Monogr Oral Sci. 2011;22:97–114.
19. Harish V, Tewari D, Gaur M, et al. Review on nanoparticles and nanostructured materials: bioimaging, biosensing, drug delivery, tissue engineering, antimicrobial, and agro-food applications. Nanomaterials. 2022;12(3):457. doi:10.3390/nano12030457
20. Naguib G, Maghrabi AA, Mira AI, Mously HA, Hajjaj M, Hamed MT. Influence of inorganic nanoparticles on dental materials’ mechanical properties. A narrative review. BMC Oral Health. 2023;23(1):897. doi:10.1186/s12903-023-03652-1
21. Sezer N, Evis Z, Kayhan SM, Tahmasebifar A, Koç M. Review of magnesium-based biomaterials and their applications. J Magnesium Alloys. 2018;6(1):23–43. doi:10.1016/j.jma.2018.02.003
22. Amukarimi S, Mozafari M. Biodegradable magnesium‐based biomaterials: an overview of challenges and opportunities. Med Comm. 2021;2(2):123–144. doi:10.1002/mco2.59
23. Cai L, Chen J, Liu Z, Wang H, Yang H, Ding W. Magnesium oxide nanoparticles: effective agricultural antibacterial agent against ralstonia solanacearum. Front Microbiol. 2018;9:790. doi:10.3389/fmicb.2018.00790
24. Arass J, Fadil A. The effect of magnesium oxide nanoparticles on the antibacterial and antibiofilm properties of glass-ionomer cement. Heliyon. 2019;5(10). doi:10.1016/j.heliyon.2019.e02568
25. Bhushan J, Maini C. Nanoparticles: a promising novel adjunct for dentistry. Indian J Dent Sci. 2019;11:167–173. doi:10.4103/IJDS.IJDS_26_19
26. Vidic J, Stankic S, Haque F, et al. Selective antibacterial effects of mixed ZnMgO nanoparticles. J Nanopart Res. 2013;15(5). doi:10.1007/s11051-013-1595-4
27. Hamalaw SJ, Kareem FA, Noori AJ. Dispersion and demineralization inhibition capacity of novel magnesium oxide nanoparticles varnish on enamel surfaces against streptococcus mutans (an In Vitro Study). Coatings. 2023;13(6):1018. doi:10.3390/coatings13061018
28. Shahmoradi M, Hunter N, Swain M. Efficacy of fluoride varnishes with added calcium phosphate in the protection of the structural and mechanical properties of enamel. Biomed Res Int. 2017;2017:7834905. doi:10.1155/2017/7834905
29. Haghgoo R, Saderi H, Eskandari M, Haghshenas H, Rezvani M. Evaluation of the antimicrobial effect of conventional and nanosilver-containing varnishes on oral streptococci. J Dentist. 2014;15(2):57–62.
30. Kis VK, Sulyok A, Hegedűs M, Kovács I, Rózsa N, Kovács Z. Magnesium incorporation into primary dental enamel and its effect on mechanical properties. Acta Biomater. 2021;120:104–115. doi:10.1016/j.actbio.2020.08.035
31. Pradhan S, Hedberg J, Blomberg E, Wold S, Odnevall Wallinder I. Effect of sonication on particle dispersion, administered dose and metal release of non-functionalized, non-inert metal nanoparticles. J Nanopart Res. 2016;18(9):285. doi:10.1007/s11051-016-3597-5
32. Medicom safety data sheet. Duraflor varnish; 2017.
33. De Luca MP, Franca JR, Macedo FAFF, et al. Propolis varnish: antimicrobial properties against cariogenic bacteria, cytotoxicity, and sustained-release profile. Biomed Res Int. 2014;2014:1–6. doi:10.1155/2014/348647
34. CLSI. Performance Standards for Antimicrobial Susceptibility Testing.
35. Balouiri M, Sadiki M, Ibnsouda SK. Methods for in vitro evaluating antimicrobial activity: a review. J Pharm Anal. 2016;6(2):71–79. doi:10.1016/j.jpha.2015.11.005
36. Galvão de LCC, Furletti VF, Bersan SMF, et al. Antimicrobial activity of essential oils against streptococcus mutans and their antiproliferative effects. Evid Based Complement Alternat Med. 2012;2012:751435. doi:10.1155/2012/751435
37. Brugger SD, Baumberger C, Jost M, Jenni W, Brugger U, Mühlemann K. Automated Counting of Bacterial Colony Forming Units on Agar Plates. PLoS One. 2012;7(3):e33695. doi:10.1371/journal.pone.0033695
38. O’Toole GA. Classic spotlight: plate counting you can count on. J Bacteriol. 2016;198(23):3127. doi:10.1128/JB.00711-16
39. Mohanta YK, Biswas K, Jena SK, Hashem A, Abd_Allah EF, Mohanta TK. Anti-biofilm and antibacterial activities of silver nanoparticles synthesized by the reducing activity of phytoconstituents present in the Indian medicinal plants. Front Microbiol. 2020;2020:11.
40. Lembke C, Podbielski A, Hidalgo-Grass C, Jonas L, Hanski E, Kreikemeyer B. Characterization of biofilm formation by clinically relevant serotypes of group a streptococci. Appl Environ Microbiol. 2006;72(4):2864–2875. doi:10.1128/AEM.72.4.2864-2875.2006
41. de Castro Melo P, Ferreira LM, Filho AN, Zafalon LF, Vicente HIG, de Souza V. Comparison of methods for the detection of biofilm formation by Staphylococcus aureus isolated from bovine subclinical mastitis. Braz J Microbiol. 2013;44(1):119–124. doi:10.1590/S1517-83822013005000031
42. Alnusayri MO, Sghaireen MG, Mathew M, Alzarea B, Bandela V. Shade selection in esthetic dentistry: a review. Cureus. 2022;14(3):e23331. doi:10.7759/cureus.23331
43. Derdilopoulou FV, Zantner C, Neumann K, Kielbassa AM. Evaluation of visual and spectrophotometric shade analyses: a clinical comparison of 3758 teeth. Int J Prosthodont. 2007;20(4):414–416.
44. Odaira C, Itoh S, Ishibashi K. Clinical evaluation of a dental color analysis system: the crystaleye spectrophotometer®. J Prosthod Res. 2011;55(4):199–205. doi:10.1016/j.jpor.2010.12.005
45. Daas I, Badr S, Osman E. Comparison between fluoride and nano-hydroxyapatite in remineralizing initial enamel lesion: an in vitro study. J Contemp Dental Pract. 2018;19(3):306–312. doi:10.5005/JP-JOURNALS-10024-2258
46. Yu OY, Mei ML, Zhao IS, Li QL, Lo ECM, Chu CH. Remineralisation of enamel with silver diamine fluoride and sodium fluoride. Dent. Mater. 2018;34(12):e344–e352. doi:10.1016/j.dental.2018.10.007
47. Amaechi BT. Protocols to study dental caries in vitro: pH cycling models. Methods Mol Biol. 2019;1922:379–392. doi:10.1007/978-1-4939-9012-2_34
48. Loveren van C, Buijs JF, Buijs MJ, Cate ten JM. Protection of bovine enamel and dentine by chlorhexidine and fluoride varnishes in a bacterial demineralization model. CRE. 1996;30(1):45–51. doi:10.1159/000262136
49. Prabhakar A, Kurthukoti A, Gupta P. Cariogenicity and acidogenicity of human milk, plain and sweetened bovine milk: an in vitro study. J Clin Pediatr Dent. 2010;34(3):239–247. doi:10.17796/jcpd.34.3.lk08l57045043444
50. Kanduti D, Sterbenk P, Artnik B. Fluoride: a review of use and effects on health. Materia Socio Medica. 2016;28(2):133–137. doi:10.5455/msm.2016.28.133-137
51. Martínez-Mier EA. Fluoride: its metabolism, toxicity, and role in dental health. J Evid Based Complementary Altern Med. 2012;17(1):28–32. doi:10.1177/2156587211428076
52. FDA U, Food and Drug Administration. CFR-Code of Federal Regulations Title 21. Washington, DC, USA: US Food and Drug Administration; 2018.
53. Haghshenas L, Amini A, Bashir Bahati A, Rahimi G. In vitro antibacterial biofilm effect of magnesium oxide nanoparticles on streptococcus mutans. Micro Nano Biomed. 2016;1(1). doi:10.15412/j.mnb.05010104
54. Weyant RJ, Tracy SL, Anselmo T, et al. Topical fluoride for caries prevention. J Am Dent Assoc. 2013;144(11):1279–1291. doi:10.14219/jada.archive.2013.0057
55. Ghada N, Khaled H, Ali H, et al. Zein based magnesium oxide nanoparticles: assessment of antimicrobial activity for dental implications. Pak J Pharm Sci. 2018;2018:245–250.
56. Rodríguez-Hernández AP, Vega-Jiménez AL, Vázquez-Olmos AR, Ortega-Maldonado M, Ximenez-Fyvie LA. Antibacterial properties in vitro of magnesium oxide nanoparticles for dental applications. Nanomaterials. 2023;13(3):502. doi:10.3390/nano13030502
57. Nguyen NYT, Grelling N, Wetteland CL, Rosario R, Liu H. Antimicrobial activities and mechanisms of magnesium oxide nanoparticles (nMgO) against pathogenic bacteria, yeasts, and biofilms. Sci Rep. 2018;8(1):16260. doi:10.1038/s41598-018-34567-5
58. Tang ZX, Lv BF. MgO nanoparticles as antibacterial agent: preparation and activity. Braz J Chem Eng. 2014;31(3):591–601. doi:10.1590/0104-6632.20140313s00002813
59. Schmitz JE, Teepe JD, Hu Y, Smith CE, Fajardo RJ, Chun YHP. Estimating mineral changes in enamel formation by Ashing/BSE and MicroCT. J Dent Res. 2014;93(3):256–262. doi:10.1177/0022034513520548
60. Walsh T, Macey R, Ricketts D, et al. Enamel caries detection and diagnosis: an analysis of systematic reviews. J Dent Res. 2022;101(3):261–269. doi:10.1177/00220345211042795
61. Pitts NB, Zero DT, Marsh PD, et al. Dental caries. Nature Rev Dis Prim. 2017;3(1):17030. doi:10.1038/nrdp.2017.30
62. Kidd EA, Fejerskov O. Essentials of Dental Caries. Oxford University Press; 2016.
63. Aspinall SR, Parker JK, Khutoryanskiy VV. Oral care product formulations, properties and challenges. Colloids Surf B. 2021;200:111567. doi:10.1016/j.colsurfb.2021.111567
64. Featherstone JDB. Dental caries: a dynamic disease process. Australian Dental Journal. 2008;53(3):286–291. doi:10.1111/j.1834-7819.2008.00064.x
65. Hamalaw SJ, Kareem FA, Gul SS. Association of dental and gingival health status with level of salivary characteristics and Streptococcus mutans in children. J Dent Sci. 2021;16(2):744–750. doi:10.1016/j.jds.2020.08.006
66. Gao X, Jiang S, Koh D, Hsu CYS. Salivary biomarkers for dental caries. Periodontology. 2016;70(1):128–141. doi:10.1111/prd.12100
67. Epple M, Meyer F, Enax J. A critical review of modern concepts for teeth whitening. Dent J. 2019;7(3):79. doi:10.3390/dj7030079
68. Abdallah MN, Eimar H, Bassett DC, et al. Diagenesis-inspired reaction of magnesium ions with surface enamel mineral modifies properties of human teeth. Acta Biomater. 2016;37:174–183. doi:10.1016/j.actbio.2016.04.005
69. Lata S, Varghese NO, Varughese JM. Remineralization potential of fluoride and amorphous calcium phosphate-casein phospho peptide on enamel lesions: an in vitro comparative evaluation. J Conserv Dent. 2010;13(1):42–46. doi:10.4103/0972-0707.62634
70. Tafaroji R, Biria M, Ameri F, Torabzadeh H, Qahari P, Akbarzadeh Baghban A. Effect of two fluoride varnishes on the color stability of three resin-based restorative materials: an in vitro study. J Invest Clin Dent. 2016;7(4):355–360. doi:10.1111/jicd.12172
71. Autio-Gold JT, Barrett AA. Effect of fluoride varnishes on color stability of esthetic restorative materials. Oper Dent. 2004;29(6):636–641.
72. Warren DP, Henson HA, Chan JT. Dental hygienist and patient comparisons of fluoride varnishes to fluoride gels. J Dent Hyg. 2000;74(2):94–101.
© 2024 The Author(s). This work is published and licensed by Dove Medical Press Limited. The full terms of this license are available at https://www.dovepress.com/terms.php and incorporate the Creative Commons Attribution - Non Commercial (unported, v3.0) License.
By accessing the work you hereby accept the Terms. Non-commercial uses of the work are permitted without any further permission from Dove Medical Press Limited, provided the work is properly attributed. For permission for commercial use of this work, please see paragraphs 4.2 and 5 of our Terms.