Back to Journals » Medical Devices: Evidence and Research » Volume 17
Mechanical Improvement of Gas Monitoring System in Monoplace Hyperbaric Chamber to Advance the Safety and Efficacy
Authors Lee HY , Lee Y, Kim H , Paik JH
Received 20 February 2024
Accepted for publication 12 June 2024
Published 20 June 2024 Volume 2024:17 Pages 217—227
DOI https://doi.org/10.2147/MDER.S465022
Checked for plagiarism Yes
Review by Single anonymous peer review
Peer reviewer comments 2
Editor who approved publication: Dr Scott Fraser
Hee Young Lee,1,* Yoonsuk Lee,1,2,* Hyun Kim,1,2 Jin Hui Paik3
1Department of Emergency Medicine, Yonsei University Wonju College of Medicine, Wonju, Gangwon State, Republic of Korea; 2Hyperbaric Oxygen Therapy Center, Wonju Severance Christian Hospital, Wonju, Gangwon State, Republic of Korea; 3Department of Emergency Medicine, Inha University College of Medicine, Incheon, Republic of Korea
*These authors contributed equally to this work
Correspondence: Hyun Kim, Department of Emergency Medicine, Yonsei University Wonju College of Medicine, 26426 Ilsan-ro, Wonju, Gangwon State, Republic of Korea, Tel +82 33 741 1613, Fax +82 33 742 3030, Email [email protected]
Introduction: A Monoplace hyperbaric chamber delivers oxygen to the patient’s tissues through breathing. Gas monitoring inside the chamber is important because oxygen (O2) is consumed, and carbon dioxide (CO2) is increased because treatment is performed in a closed volume. This study aimed to advance the safety and efficacy of the monoplace hyperbaric chamber (MHC) through mechanical improvement in a gas monitoring system (GMS).
Methods: First, as the oxygen supply method was changed to the direction of the patient’s face, it was compared the values of O2, CO2, humidity, and temperature were measured in the MHC and the GMS when operating at 2.0 atmosphere absolute (ATA) and 3.0 ATA. Second, to evaluate the effects of variables across measuring time, it was analyzed in a 3-way repeated measure ANOVA (10 min.× 20 min.× 30 min.). Lastly, the values before and after the optimization of the MHC were compared by applying a cooler to prevent temperature rise inside the MHC.
Results: In 2.0 ATA, the average humidity was higher in the MHC than in the GMS (p< 0.001). Also, the average temperature was lower in the MHC than in the GMS (p< 0.001). In 3.0 ATA, the average CO2 and humidity were higher in the MHC than in the GMS, respectively (p< 0.001, p=0.004). The 3-way repeated measures ANOVA revealed a significant difference in most main and interacted factors (p< 0.05). O2 and temperature, comparing before and after MHC optimization, revealed a significant difference (p< 0.05).
Conclusion: Few studies have verified safety and effectiveness by evaluating the pressure, oxygen concentration, etc. of a monoplace hyperbaric chamber. Further research is expected to verify the effectiveness of providing comfort to patients receiving hyperbaric oxygen treatment and increase the treatment effect.
Keywords: monoplace hyperbaric chamber, gas monitoring system, mechanical improvement, safety and efficacy
Graphical Abstract:
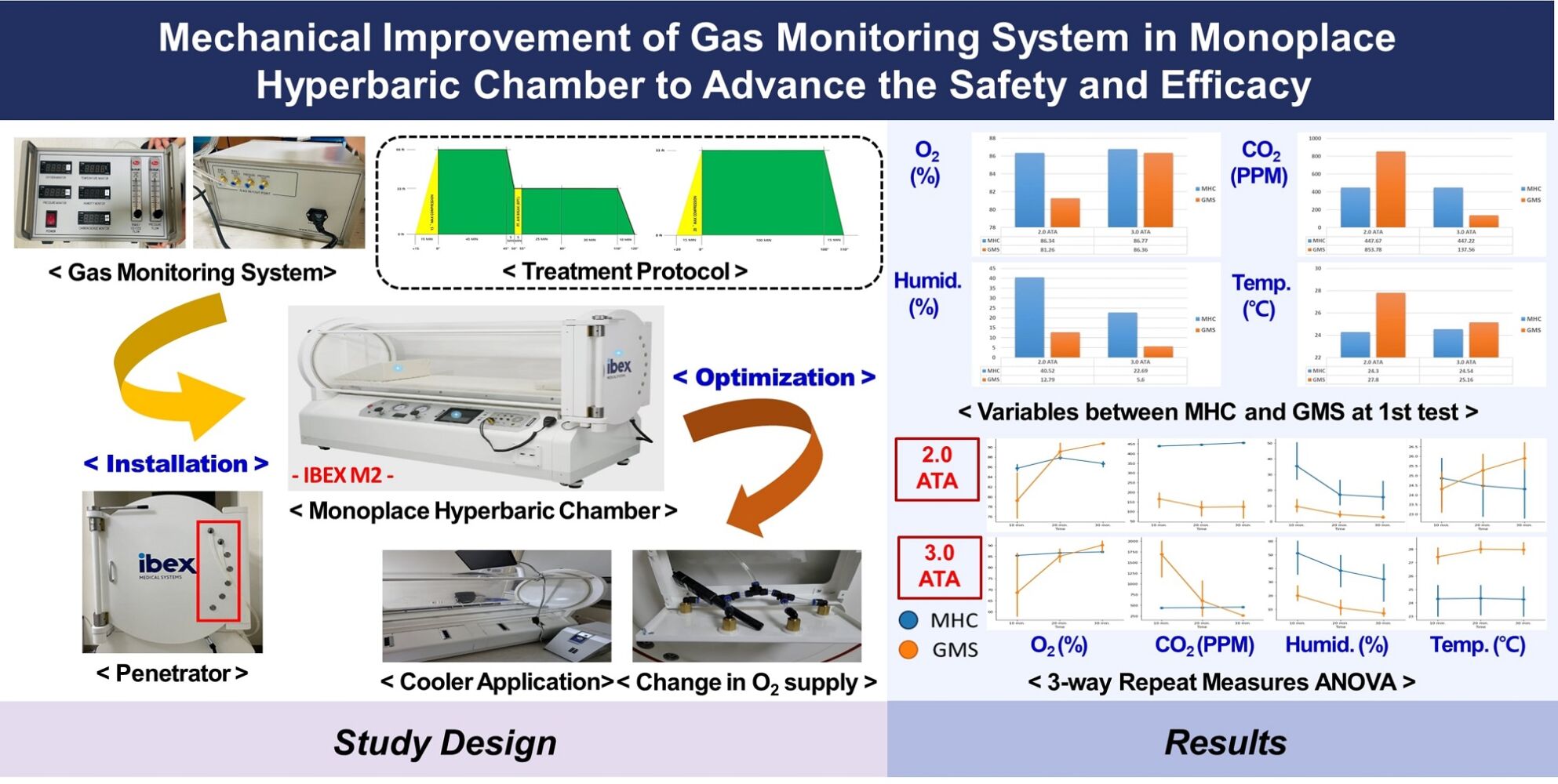
Introduction
In humans, oxygen plays an important role in providing energy and maintaining life and function.1 Oxygen in the blood is divided into combined and dissolved oxygen. Since oxygen has low solubility at atmospheric pressure, most of the oxygen during respiration is combined with hemoglobin in the blood to become bound oxygen, and the remaining oxygen is released into the air through gas exchange or dissolved in plasma (dissolved oxygen).2 Combined oxygen, which is bigger than dissolved oxygen, passes smoothly through capillaries to each organ and cell that requires it. When blood vessels are abnormally narrowed due to unhealthy diet, smoking, drinking, obesity, etc., or when blood obesity increases due to diabetes or hyperlipidemia, the bound oxygen combined with hemoglobin cannot pass through capillaries. A variety of diseases and defects are associated with a lack of oxygen supply (hypoxia), which can lead to severe disability and even death.3
The first report on the therapeutic use of hyperbaric oxygen therapy (HBOT) was published in 1879 by Fontaine, a French surgeon who believed that pressurized oxygen chambers could help with anesthesia.4 HBOT is used to treat patients by supplying 100% oxygen at more than twice the atmospheric pressure. This therapy is commonly applied to treat cesarean disease, gas poisoning, wounds, and burns. It is known to be effective in decompression sickness (DCS), carbon monoxide (CO) poisonings, diabetic foot, etc. Research on indications is being actively conducted.5,6 Also, the Undersea and Hyperbaric Medicine Society (UHMS) in the United States provides guidelines and recommendations for the use of HBOT in various medical conditions. For clinical efficacy, it was specified that the pressure must be greater than or equal to 1.4 atmosphere absolute (ATA), and in clinical practice, pressures applied usually range from 2.0 to 3.0 ATA.7
Hyperbaric chambers are used in high-pressure environments where a pressure of at least two or more times atmospheric pressure is set and maintained. It is treated for 45 to 300 minutes in a pressurized environment with 1 atm or 2 atm pressure depending on the purpose or severity. According to recent studies on the indications of HBOT, it is expected to have positive effects in the treatment of hypoxia, oxidative stress, inflammation-induced brain damage, congenital cerebral palsy, stroke, autism, Alzheimer’s disease, Parkinson’s syndrome, and chronic Lyme disease, and cancers.8 Although no clear evidence for the effects and mechanism of HBOT on the treatment of intractable diseases has been discovered, it has shown favorable results in many clinical cases. According to the number of users, it was classified into monoplace and multiplace chamber.9 Monoplace chamber delivers oxygen to the patient’s tissues through breathing, and multiplace chamber delivers oxygen to the patient’s tissues through a breathing aid such as a mask.
In the case of a monoplace chamber, gas monitoring inside the hyperbaric chamber is important because oxygen is consumed, and carbon dioxide is increased because treatment is performed in a closed volume. Values may vary slightly depending on where the oxygen and carbon dioxide concentrations are measured in the hyperbaric chamber. In general, 100% oxygen is supplied during HBOT. Still, the oxygen concentration in the hyperbaric chamber may not be constant because a difference in oxygen partial pressure may occur according to a pressure change. The goal is to increase the effectiveness of HBOT by measuring the concentration change of these internal gases and supplying low carbon and high oxygen to the patient. In addition, the temperature and humidity are fluctuated together according to the change in pressure in the closed treatment device, and when the pressure rises, the temperature and humidity also rise together. It is important to measure the changing pressure and maintain the temperature and humidity according to the pressure change in order to provide the optimal treatment environment to the patient and to become an accurate HBOT.
Breathing plays a critical role in hyperbaric oxygen therapy (HBOT) as the efficiency of oxygen delivery to tissues is directly influenced by respiratory mechanics. Lung physiology under hyperbaric conditions differs significantly from that at normal atmospheric pressure.10 The increased pressure enhances the amount of oxygen dissolved in plasma, thereby improving oxygen availability to hypoxic tissues. Conditions in hyperbaric chambers such as pressure, temperature, and humidity must be meticulously controlled to optimize patient safety and therapeutic outcomes.11 Monitoring breathing parameters and ensuring the stability of these environmental conditions is crucial for the effectiveness of HBOT.12,13 Furthermore, understanding the impact of lung physiology in hyperbaric environments helps in designing better therapeutic protocols and improving patient care.
According to the current status of hyperbaric chamber deployment investigated by the Korean Academy of Undersea and Hyperbaric Medicine, the number of medical institutions capable of HBOT in Korea increased from 21 to 50 in 2021 compared to the first survey in 2017. The details of number of medical institutions capable of HBOT increased from 8 to 13 for tertiary general hospitals, from 3 to 17 for general hospitals, and from 10 to 20 for hospitals and other medical institutions. The number of multiplace hyperbaric chambers increased from 12 to 30, and the number of monoplace hyperbaric chambers increased from 13 to 43.14
However, to our knowledge, no study has verified safety and effectiveness by evaluating the pressure, oxygen concentration, etc. of a monoplace hyperbaric chamber. This study aims to advance the safety and efficacy of monoplace hyperbaric chamber through mechanical improvement in a gas monitoring system.
Materials and Methods
Monoplace Hyperbaric Chamber and Ethical Approval
IBEX M2 (IBEX Medical Systems Co. Ltd., Republic of Korea) was a newly developed and recently commercialized monoplace hyperbaric chamber as a medical device for testing (Figure 1). It was applied the automatic control system including the anti-barotrauma technology. This study was approved by the Institutional Review Board of Wonju Christian Hospital (IRB approval no.: CR320074) and the Clinical Research Information Service of Korea Disease Control and Prevention Agency (Trial registration no.: KCT0005974).
Development of the Gas Monitoring System and Its Connection to the Chamber
A sensor kit was developed by selecting a data recorder and a transmitter (Figure 2). The O2H-9903SD (LT Lutron Electronic Enterprise Co. Ltd., Taiwan) is the data recorder that was capable of O2, humidity, and temperature detection into the SD memory card. It has specified measurement ranges and accuracies for each oxygen, humidity, and temperature as follows; 0~30% O2±(1% reading+0.2%), 5~95% RH±3% (below 70% RH), and 0~50±0.8°C or 32~122±1.5°F. Also, the CD-100 (ELT SENSOR Corp., Republic of Korea) is the one-board type transmitter that was capable of measuring CO2 concentration. It has a specified measurement range of 0~2000 ppm and measurement accuracy of 5% ± 30 ppm. The developed gas monitoring system was connected with a hose after installing a penetrator on the door side of the monoplace hyperbaric chamber (Figure 3).
Optimization of Monoplace Hyperbaric Chamber
The sensor kit of the gas monitoring system was upgraded to increase the accuracy of the measured oxygen concentration applied to the chamber. A flow function according to the carbon monoxide concentration value was added, and an air brake system application method was configured. The oxygen supply method was changed in the direction of the patient’s face so that the patient could receive a comfortable HBOT (Figure 4A). A cooler was applied to control the temperature inside the chamber, and a smartphone remote function was used (Figure 4B).
Pressure Value Settings for the Safety and Effectiveness Evaluation of Monoplace Hyperbaric Chamber
The acute carbon monoxide poisoning treatment protocol, which is the most commonly used indication for hyperbaric oxygen treatment at the HBOT Center at Wonju Severance Christian Hospital, was applied in this study. Based on the hyperbaric oxygen treatment guidelines established by the US Navy, specialists from the Korean Society of Hyperbaric Medicine partially modified this protocol to change the pressure more slowly for 15 minutes so that patients can smoothly adapt to decompression (Figure 5).15 The 1st treatment was performed using 3.0 ATA and 2.0 ATA for 135 minutes. Before starting the HBOT session, it was prepared for max compression for 15 minutes and then performed initial compression for 45 minutes to 3.0 ATA. Then, the pressure was reduced from 3.0 ATA to 2.0 ATA for 5 minutes, and the air brake was maintained for 5 minutes. Next, it was followed by 2.0 ATA for 55 minutes and ended with depressurization for 10 minutes (Figure 5A). Other than the 1st treatment was performed using 2.0 ATA for a total of 110 minutes. Before starting the HBOT session, it was prepared for max compression for 20 minutes and then performed initial compression for 100 minutes to 2.0 ATA. After that, it was decompressed for 15 minutes (Figure 5B).
Study Design
When the monoplace hyperbaric chamber was operated at 2.0 ATA and 3.0 ATA, the values of oxygen, carbon dioxide, humidity, and temperature were measured in the chamber and gas monitoring system, respectively. In 2.0 ATA, the subject entered the chamber, but in 3.0 ATA, the subject did not enter the chamber to prevent a situation in which the subject could be in danger due to high pressure. The data has measured a total of three times during the chamber operating at 10 minutes, 20 minutes, and 30 minutes after pressurization, and this sequence was repeated three times. Finally, after optimizing the monoplace hyperbaric chamber based on the descriptive results of variables according to air pressure conditions, the second test was performed again using the same process.
Statistical Analysis
Statistical analysis was performed using IBM SPSS Statistics Version 27.0 (IBM Corp., Armonk, NY, USA). In this study, the values measured in the monoplace hyperbaric chamber and a gas monitoring system were compared. Comparisons among the measured data between the monoplace hyperbaric chamber and a gas monitoring system were expressed as means with the standard deviations for continuous variables. Differences were assessed using the independent t-test or Mann–Whitney U-test for continuous variables and the χ2 test or Fisher exact test for categorical variables. It was interpreted as statistically significant when the p-value was less than 0.05. To evaluate changes in the values measured at 10, 20, and 30 minutes after pressurization, a 3-way repeated measures analysis of variance (ANOVA) was performed. Assumptions of sphericity were examined, and when violated, the Greenhouse-Geisser correction was applied.
Results
In the air pressure condition at 2.0 ATA, the average humidity was higher in the chamber than in the gas monitoring system (40.52±14.13 % vs 12.79±7.31 %, p<0.001). Also, the average temperature was lower in the chamber than in the gas monitoring system (24.30±0.99 °C vs 27.80±0.54 °C, p<0.001). In the air pressure condition at 3.0 ATA, the average carbon dioxide was higher in the chamber than in the gas monitoring system (447.22±7.85 PPM vs 137.56±43.70 PPM, p<0.001). Also, the average humidity was higher in the chamber than in the gas monitoring system (22.69±13.14 % vs 5.60±3.93 %, p=0.004) (Table 1).
![]() |
Table 1 Descriptive Analysis (Mean, SD) of Variables According to Air Pressure at the First Test |
The 3-way repeated measures ANOVA showed the significance of the device (D), measuring time (T), and interactions between the device and measuring time (DxT) on the effects of variables across measuring time (Table 2). In 2.0 ATA air pressure conditions, significant main effects were observed for the time in O2, the device in CO2, and the time in humidity (p<0.001). Also, the time in CO2, the device in humidity, the device in temperature, and the time in temperature revealed significant main effects respectively (p=0.003, p=0.029, p=0.009, p=0.010). Significant interactions were observed between device and time in O2, CO2, and temperature, respectively (p=0.001, p=0.002, p=0.003). In 3.0 ATA air pressure conditions, significant main effects were observed for the device in CO2, the time in humidity, and the time in temperature (p<0.001). Also, the time in O2, the time in CO2, and the device in humidity revealed significant main effects respectively (p=0.008, p=0.016, p=0.047). Significant interactions were observed between the device and time in CO2, and temperature (p<0.001). Furthermore, the interaction between the device and time in O2, and humidity revealed significant effects respectively (p=0.020, p=0.010).
![]() |
Table 2 Results of 3-Way Repeated Measures ANOVA for the Effects of Variables Across Measuring Time |
Descriptive statistics for variables according to air pressure conditions before and after optimization of the monoplace hyperbaric chamber (mean±SD) are presented (Table 3). In 2.0 ATA air pressure condition, the paired samples t-test comparing O2 before and after optimization of the monoplace hyperbaric chamber revealed a significant difference (t(df)=−14.133, p=0.040). The p-value of less than 0.001 indicated that the difference between temperature before and after optimization was statistically significant. In 3.0 ATA air pressure condition, the paired samples t-test comparing O2, humidity, and temperature before and after optimization of the monoplace hyperbaric chamber revealed a significant difference, respectively (t(df)=−7.467, p=0.038 / t(df)=−10.800, p=0.002 / t(df)=1.767, p=0.013).
![]() |
Table 3 Comparison of Variables Before and After Optimization of the Monoplace Hyperbaric Chamber |
Discussion
This study aimed to advance the safety and efficacy of a monoplace hyperbaric chamber through mechanical improvement in a gas monitoring system. The developed gas monitoring system was connected with a hose after installing a penetrator on the door side of the chamber. As the oxygen supply method was changing toward the direction of the patient’s face, it made the patient receive a comfortable HBOT while increasing the accuracy of the measured oxygen concentration applied to the chamber. Sechrist et al reported that it described the use of the HBO O2 Smart Guide, an Excel-based program that could calculate hyperbaric oxygen concentrations, given a set of initial conditions. In contrast, it was described how the program was validated by measuring the oxygen in-chamber, using 4 different monoplace chamber models, and employing 2 types of oxygen purge profiles.16 Sumbul and Yuzer presented that the integration of MEMS-based diagnostic tools for respiratory monitoring within monoplace hyperbaric chambers offers significant advantages. The detection of respiratory rate (RR), tidal volume capacity (TVC), and forced vital capacity (FVC) using a MEMS-based acceleration sensor can provide real-time, accurate respiratory measurements. These tools record the acceleration data of diaphragm movements in three axes during respiration, ensuring precise monitoring of the patient’s respiratory parameters during HBOT sessions.13 Also, they reported another study involving five subjects (three healthy and two with COPD) demonstrated the reliability of this MEMS-based system. It was able to measure and record respiratory parameters accurately, with results closely matching those from a medical spirometer. Such accuracy is crucial in hyperbaric environments where precise oxygen delivery and patient monitoring are essential for safety and treatment efficacy.10 Furthermore, the development of a practical method for estimating air volume using acceleration data from diaphragm movements reinforces the potential of these advanced diagnostic tools. By providing real-time data, clinicians can dynamically adjust treatment parameters, enhancing the safety and effectiveness of HBOT. This integration aligns perfectly with the goal of mechanical improvement in gas monitoring systems in monoplace hyperbaric chambers, ensuring better patient outcomes.12
Table 1 compares the values measured in the existing chamber with those measured in the newly developed gas monitoring system. In this study, the average humidity was higher in the chamber than in the gas monitoring system in the air pressure condition at 2.0 ATA and the average temperature was lower in the chamber than in the gas monitoring system in the air pressure condition at 3.0 ATA. The difference in measured values between the hyperbaric oxygen chamber and gas monitoring system at 2.0 ATA and 3.0 ATA could be caused by a variety of factors. First, the difference in measured values could be caused by the pressure differences. Similarly, Ay et al found that increased pressure in hyperbaric chambers could lead to increased levels of humidity due to reduced ventilation and moisture from medical gases.17 Second, the difference in measured values could be caused by the ventilation differences. The hyperbaric chamber might have different ventilation settings than the gas monitoring system. At 2.0 ATA, where subjects entered the chamber, the CO2 levels were notably higher. This elevation in CO2 levels can be attributed to human respiration, a natural process that results in the production of carbon dioxide as a metabolic byproduct. The relationship between pressure and CO2 levels is intricate but can be elucidated by considering Henry’s Law, which states that the solubility of a gas (such as CO2) in a liquid (such as blood) is directly proportional to the pressure of that gas above the liquid.18 Thus, at 2.0 ATA, the higher pressure and increased CO2 production from respiration likely contributed to the elevated CO2 readings. Conversely, at 3.0 ATA where no subjects were present due to safety concerns associated with high pressure, the CO2 levels were lower. Without human respiration as a significant source of CO2, the primary mechanisms contributing to CO2 levels would be environmental factors and any potential off-gassing from materials within the chamber. The measured differences in CO2 levels between the two pressure conditions can be attributed to the presence or absence of individuals and their corresponding metabolic activities, particularly respiration. Understanding the relationship between pressure and CO2 levels, along with the influence of human presence, is considered crucial for interpreting gas monitoring system data accurately in hyperbaric environments.19 Lastly, the difference in measured values could be caused by the temperature differences. The hyperbaric chamber might be at a different temperature than the gas monitoring system, which could affect the readings of humidity and temperature. At 2.0 ATA, the lower average temperature readings in the hyperbaric chamber might be due to the lower ambient temperature in the chamber.20
Table 2 presents the significance of the device (D), measuring time (T), and interactions between the device and measuring time (DxT) on the effects of variables across measuring time by the 3-way repeated measures ANOVA. In summary, under 2.0 ATA air pressure conditions, the 3-way repeated measures ANOVA revealed significant main effects of the time in O2, the device in CO2, the time in CO2, the device in humidity, the time in humidity, the device in temperature, and the time in temperature, as well as an interaction between device and time in O2, CO2, and temperature. Under 3.0 ATA air pressure conditions, significant main effects were observed for the device in CO2, the time in humidity, and the time in temperature. Also, the time in O2, the time in CO2, and the device in humidity revealed significant main effects. Significant interactions were observed between the device and time in all variables revealing significant effects. Although we could not find any previous studies that measured and monitored O2, CO2, humidity, and temperature in the monoplace hyperbaric chamber like this study, Gertner et al reported a time-dependent study using the chamber using a 3-way repeated measures ANOVA methodology. There were several potential confounding factors identified that may have affected the observed results to determine the efficacy of various drugs to decrease the incidence of Eustachian Tube Dysfunction in Divers.21
Table 3 compared the values before and after optimization of a monoplace hyperbaric chamber by applying a cooler to prevent temperature rise inside the chamber. In 2.0 ATA air pressure condition, the paired samples t-test comparing O2, and temperature before and after optimization of the monoplace hyperbaric chamber revealed a significant difference. In 3.0 ATA air pressure condition, the paired samples t-test comparing O2, humidity, and temperature before and after optimization of the monoplace hyperbaric chamber revealed a significant difference. In this study, O2 was increased after optimization of the monoplace hyperbaric chamber. Similarly, the prevailing perspective suggests that the effectiveness of HBOT is positively correlated with increasing oxygen concentrations. In HBOT, patients are exposed to elevated atmospheric pressures while breathing pure oxygen, aiming to augment the amount of dissolved oxygen in the bloodstream. Although some proponents argue that higher oxygen concentration under hyperbaric oxygen conditions improves oxygen supply, accelerates the healing process, reduces inflammation, and improves the overall treatment effect, what actually increases the effectiveness of hyperbaric oxygen treatment is the medical staff’s This can be attributed to careful consideration of factors, adherence to established safety guidelines, and awareness of the potential risks associated with excessive oxygen levels, including oxygen toxicity. Also, the temperature was decreased after optimization of the monoplace hyperbaric chamber. HBOT is often associated with providing a comfortable therapeutic environment, and the temperature during HBOT sessions plays a crucial role in enhancing patient comfort. As the ambient pressure increases within the hyperbaric chamber, the temperature is carefully controlled to ensure the well-being and comfort of the patient. The controlled temperature not only contributes to the overall comfort but also plays a role in mitigating potential discomfort that may arise from the increased pressure. Maintaining a cooler temperature within the chamber is often considered beneficial, as it can alleviate any potential feelings of warmth or claustrophobia associated with the enclosed environment. Additionally, a cooler environment may help minimize the risk of overheating, especially during longer treatment sessions. Overall, careful control of the temperature of the monoplace hyperbaric chamber is a key aspect of ensuring the safety of patients undergoing HBOT and providing them with a positive and comfortable experience while receiving treatment.22
Conclusion
Despite the limitations in this study, to our knowledge, no study has verified the safety and effectiveness of the monoplace hyperbaric chamber by evaluating its pressure and oxygen concentration. Therefore, this study monitored the conditions inside the hyperbaric oxygen chamber. This study was meaningful in that it attempted to develop a gas monitoring system that operates and improves its safety and efficacy. Lastly, based on the results of this study, additional research is needed to verify whether hyperbaric oxygen treatment actually increases treatment effectiveness and provides comfort to patients receiving hyperbaric oxygen treatment.
Acknowledgments
We would like to thank the medical staff (doctors, nurses, and emergency medical technicians) who tested the monoplace hyperbaric chamber at the Hyperbaric Oxygen Therapy Center at Wonju Severance Christian Hospital. We also thank the engineers (IBEX Medical Systems Co. Ltd.) for their technical support.
Funding
This research was supported by the Korea Medical Device Development Fund grant funded by the Korea government (the Ministry of Science and ICT, the Ministry of Trade, Industry and Energy, the Ministry of Health & Welfare, Republic of Korea, the Ministry of Food and Drug Safety) (Project Number: RS-2020-KD000247). It was also supported by a grant from the Korea Health Technology R&D Project through the Korea Health Industry Development Institute (KHIDI), funded by the Ministry of Health & Welfare, Republic of Korea (No. HR21C0885).
Disclosure
The authors report no conflicts of interest in this work.
References
1. Clauss W, Clauss C. Humanbiologie Kompakt.
2. Widmaier E, Raff H, Strang KT. Vander’s Human Physiology. McGraw-Hill US Higher Ed USE; 2022.
3. Jain KK. Textbook of Hyperbaric Medicine.
4. Jhj I, Morsch JH, Rendell‐Baker L. The historical perspective of hyperbaric therapy. Ann N Y Acad Sci. 1965;117(2):651–670. doi:10.1111/j.1749-6632.1964.tb56312.x
5. Leach RM, Rees PJ, Wilmshurst P. Hyperbaric oxygen therapy. BMJ. 1998;317(7166):1140–1143. doi:10.1136/bmj.317.7166.1140
6. Tibbles PM, Edelsberg JS. Hyperbaric-oxygen therapy. N Engl J Med. 1996;334(25):1642–1648. doi:10.1056/NEJM199606203342506
7. Undersea and Hyperbaric Medical Society. Indications for hyperbaric oxygen therapy. Available from: https://uihc.org/educational-resources/indications-hyperbaric-oxygen-therapy.
8. Al-Waili NS, Butler GJ, Beale J, et al. Hyperbaric oxygen in the treatment of patients with cerebral stroke, brain trauma, and neurologic disease. Adv Ther. 2005;22(6):659–678. doi:10.1007/BF02849960
9. Mayer R, Hamilton-Farrell MR, van der Kleij AJ, et al. Hyperbaric oxygen and radiotherapy. Strahlenther Onkol. 2005;181(2):113. doi:10.1007/s00066-005-1277-y
10. Sümbül H, Yüzer AH. Development of diagnostic device for COPD: a MEMS based approach. Int J Comput Sci Network Secur. 2017;17(7):196–203.
11. Demir AE, Ilbasmis S, Toklu AS. A COVID-19 infection incidentally detected during hyperbaric oxygen treatment and preventive measures for COVID-19 transmission in a multiplace hyperbaric chamber. Diving Hyperbaric Med. 2022;52(1):58. doi:10.28920/dhm52.1.58-62
12. Sümbül H, Yüzer AH. Estimating the value of the volume from acceleration on the diaphragm movements during breathing. J Eng Sci Technol. 2018;13(5):1205–1221.
13. Sümbül H, Yüzer AH. Measuring of diaphragm movements by using iMEMS acceleration sensor. In
14. Lee SM, Heo T, Kim GW, et al. Current status and development direction of hyperbaric medicine in Korea. J Korean Med Assoc. 2022;65(4):232–238. doi:10.5124/jkma.2022.65.4.232
15. Kessler L, Bilbault P, ORTega F, et al. Hyperbaric oxygenation accelerates the healing rate of nonischemic chronic diabetic foot ulcers: a prospective randomized study. Diabetes Care. 2003;26(8):2378–2382. doi:10.2337/diacare.26.8.2378
16. Sechrist JR, Warriner III RA, Weninger AE, et al. Validation of hyperbaric oxygen treatment software for use with monoplace chambers. Undersea Hyperb Med. 2008;35(3):219.
17. Ay H, Salihoglu M, Altundag A, et al. The effect of hyperbaric conditions on olfactory functions. Undersea Hyperb Med. 2014;41(3):203–207.
18. Edwards ML. Hyperbaric oxygen therapy. Part 1: history and principles. J Veterinary Em Critical Care. 2010;20(3):284–288. doi:10.1111/j.1476-4431.2010.00535.x
19. Neuman TS, Thom SR. Physiology and medicine of hyperbaric oxygen therapy. Elsevier Health Sci. 2008.
20. American Society of Heating Refrigerating and Air-Conditioning Engineers. 2017 ASHRAE Handbook. Fundamentals. Inch-Pound Ed. Atlanta GA: ASHRAE; 2017.
21. Gertner J, Duplessis C, Fothergill D, et al. Evaluation of Drug Effects on Eustachian Tube Dysfunction in Divers. Int J Med. 2010.
22. Weaver LK, Strauss MB. Monoplace Hyperbaric Chamber Safety Guidelines. Bethesda, MD: Undersea and Hyperbaric Medical Society (UHMS); 1997.
© 2024 The Author(s). This work is published and licensed by Dove Medical Press Limited. The full terms of this license are available at https://www.dovepress.com/terms.php and incorporate the Creative Commons Attribution - Non Commercial (unported, v3.0) License.
By accessing the work you hereby accept the Terms. Non-commercial uses of the work are permitted without any further permission from Dove Medical Press Limited, provided the work is properly attributed. For permission for commercial use of this work, please see paragraphs 4.2 and 5 of our Terms.